Practice Essentials
Osteoporosis is defined as a progressive systemic skeletal disorder characterized by low bone mineral density (BMD), deterioration of the microarchitecture of bone tissue, and susceptibility to fracture. The reduction in skeletal mass results from an imbalance between bone resorption and bone formation; increased bone resorption or decreased bone formation may result in osteoporosis. The 2 most important factors that contribute to development of osteoporosis are aging and loss of gonadal function. Bone loss in women accelerates quickly during the first years following menopause. [1, 2, 3, 4, 5]
Each year, osteoporosis results in approximately 9 million fractures worldwide. Prevalence increases dramatically with age, particularly in women, rising from 2% at 50 years of age to over 25% at 80 years of age. [2]
Dual-energy x-ray absorptiometry (DEXA) detects small changes in bone mass by comparing the patient's bone density to that of healthy adults (T score) and to age-matched adults (Z score). Assigning the T score permits the early detection of osteoporosis and thus lowers the risk of either hip or spine fractures. However, the use of T scores at different sites and with different techniques has been controversial because intersite and intermodality correlation has been poor (see the images below). [6, 7, 8, 2, 9, 3, 10, 11]
Early detection of vertebral fracture may increase the diagnosis of osteoporosis. It has been reported that detection of severe osteoporosis significantly increased by 17.2% in patients who received vertical fracture assessment (VFA). [3]
Methods for BMD measurement
The most accurate way to diagnose osteoporosis is by measuring bone mass. Bone mineral density (BMD) is determined by measuring the amount of bone mineral (calcium hydroxyapatite) per unit volume of bone tissue. X-rays or gamma rays are often used to quantify BMD. In quantitative terms, BMD is the amount of calcium hydroxyapatite, or Ca10 (PO4)6 (OH)2, per unit volume of bone tissue examined. Common methods include conventional radiography, quantitative CT (QCT), single-photon absorptiometry (SPA), dual-photon absorptiometry (DPA), quantitative ultrasonography (QUS), [12] and dual-energy x-ray absorptiometry (DEXA). DEXA scans can be used to detect small changes in bone mass by comparing the patient's bone density to that of healthy adults (T score) and to age-matched adults (Z score). [8, 13, 14, 15, 16, 17, 2, 9, 3, 18, 19, 11]
A number of methods have been developed for the in vivo determination of bone density in patients at risk for osteoporosis. Two of the most frequently used methods are based on measuring the attenuation of a beam of electromagnetic radiation or ultrasound when it passes through the bone. Ultrasonic measurement of velocity through the bone has also been used to determine bone density. [20, 21, 22, 23, 24, 25, 26, 27, 28, 29, 30, 31, 32, 33]
Currently, DEXA is the most accurate and recommended method for BMD measurement. It is a sensitive technique and can detect changes in bone density only 6-12 months after a previous measurement is obtained. Density measurements of the spine or hip are used. The procedure takes approximately 20-30 minutes. The radiation exposure is low at approximately 2.5 mrem.
Bone biopsy may be useful in unusual forms of osteoporosis, such as osteoporosis in young adults. Biopsy provides information about the rate of bone turnover and the presence of secondary forms of osteoporosis, such as myeloma and systemic mastocytosis. Patients with a high turnover usually respond better to antiresorptive drugs than to other treatments. Bone turnover can also be evaluated by estimating certain biochemical markers, such as osteocalcin and deoxypyridinoline. [34] Biochemical markers can be more useful than bone density for monitoring treatment, as changes in bone density may not be detected for 2 years. [35, 36]
Recommendations for BMD testing
The National Osteoporosis Foundation (NOF) recommends bone density testing for the following groups [37] :
-
Women aged 65 years or older and men aged 70 yearsor older.
-
Postmenopausal women younger than 65 years who have at least 1 additional risk factor.
-
Men aged 50-69 years with at least 1 additional risk factor.
-
Anyone who has had a fracture after aged 50 years.
In addition, the NOF recommends patients treated with osteoporosis medicine should repeat their bone density test by central DXA every 1-2 years. [37]
In postmenopausal women being treated for osteoporosis, the Endocrine Society suggests monitoring the bone mineral density by DEXA at the spine and hip every 1-3 years to assess the response to treatment. [38]
The American Association of Clinical Endocrinologists and the American College of Endocrinology guidelines for postmenopausal osteoporosis recommend BMD testing by DXA for postmenopausal women aged 50 years or older based on their risk profile. [39]
The UK National Osteoporosis Guideline Group (NOGG) recommends bone density measurements for postmenopausal women and for men aged 50 years or older who have risk factors for fracture. Clinical risk factors include the following [40] :
-
Low body mass index (BMI)
-
History of a prior fracture at a site characteristic for osteoporosis
-
Parental history of hip fracture
-
History of smoking
-
Glucocorticoid use
-
Alcohol consumption
-
Rheumatoid arthritis
Intervention
Vertebral body stenting has been introduced as a novel percutaneous cement–augmenting technique for the treatment of vertebral body compression fractures and has produced controversy as to which technique is better—vertebral body stenting versus kyphoplasty—for the treatment of osteoporotic vertebral compression fractures. [41]
Werner et al set up a 2-armed randomized, controlled trial in patients with a total of 100 fresh osteoporotic vertebral compression fractures treated with either balloon kyphoplasty or vertebral body stenting. The purpose of this study was to clarify whether there are relevant differences between balloon kyphoplasty and vertebral body stenting with regard to perioperative and postoperative findings. The primary outcome was of the postinterventional change in the kyphotic angle on radiographs. The secondary outcomes were the maximum pressure of the balloon during inflation, radiation exposure time, perioperative complications, and cement leakage. [41] The trial concluded that there was no beneficial effect of vertebral body stenting over balloon kyphoplasty among patients with painful osteoporotic vertebral fractures with regard to kyphotic correction, cement leakage, radiation exposure time, or neurologic sequelae. Vertebral body stenting was associated with significantly higher pressures during balloon inflation and more material-related complications.
Limitations of techniques
Plain radiography is widely available but is not preferred because it is not suitable for the early detection of osteoporosis. Changes on plain radiographs can be seen only after approximately 30% of the bone is lost. However, plain radiographs are useful to rule out osteoporotic fractures and other pathology, such as myeloma. Radiation exposure for an average radiograph is approximately 50 mrem. (See the images below.)
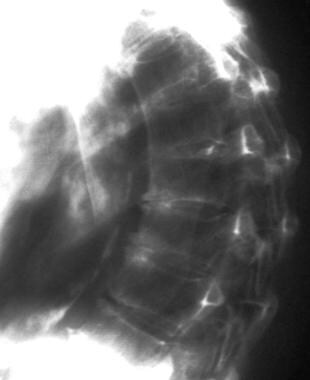
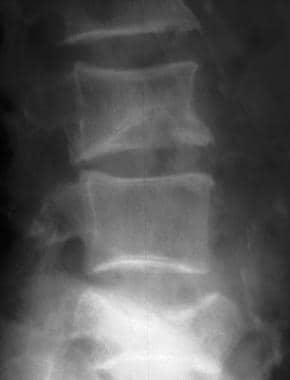
Bone-density measurements are not an effective method to monitor the response to treatment because changes in bone density may not be detected for up to 2 years. Radiation techniques to measure BMD, such as single-photon absorptiometry and DPA, have several limitations. The most important limitation is posed by the inhomogeneity of soft tissues because different components have their own attenuation coefficients. Fat has the lowest attenuation and is generally unevenly distributed in the region of BMD measurement; therefore, it has a variable affect on the accuracy of the measurement. The accuracy of density and attenuation coefficients for the bone mineral and soft-tissue components are also uncertain, though this limitation can partly be overcome with direct DPA and DXA measurement.
The accuracy of photon absorptiometry has been estimated to be 4-8% for SPA and 4-6% for DXA. However, the accuracy can be as low as 11% and is worse for lateral projections, as compared to anteroposterior (AP) projections.
SPA is used to measure forearm bone density, and it may not provide an accurate assessment of bone density of the spine or hip. The procedure takes about half an hour. Radiation exposure from SPA is approximately 5 mrem. DPA is used to measure the density of the spine or hip. The precision of DPA is acceptable for diagnosing osteoporosis but insufficient for detecting changes in individual patients. Radiation exposure from DPA is approximately 5 mrem.
Soft-tissue inhomogeneity affects the accuracy of QCT. The content of yellow marrow in the vertebrae may have a significant effect on the accuracy of BMD measurements. Machine-related artifacts, such as beam hardening, might also affect its accuracy. Overall, the value of single-energy methods is in the range of 5-15%. With 2 effective beam energies, this changes to 3-10%, but at the cost of poor precision. The precision and accuracy of QCT is good, but the radiation involved is relatively high (approximately 200-300 mrem). Therefore, QCT is not a preferred technique when other methods are available.
Ultrasound transmission is attenuated by the thickness and composition of tissues within and surrounding the bone. In trabecular bone, fatty marrow in the intertrabecular spaces influences both broadband ultrasound attenuation (BUA) and velocity. Measurements are determined by means of ultrasonography of the heel. Osteoporosis Australia's Consensus Statement [42] states that this type of measurement of bone strength lacks acceptable measurement precision and long-term stability to be recommended for use in the diagnosis of osteoporosis. If such ultrasound measurements show low bone density, the patient should be referred for DEXA because of its high accuracy and precision.
Radiography
Conventional radiographs are relatively insensitive for demonstrating osteoporosis. At least 30% of the bone mass must be lost before the loss is recognized. At this stage, the radiographic changes of generalized osteoporosis are more prominent in the axial skeleton than elsewhere. [9, 4]
In the spine, the accentuated primary trabecular pattern produces a vertically striated appearance in the vertebral bodies. Likewise, the loss of trabecular mass causes accentuation of the cortical outline, which is described as "picture framing" of the vertebral bodies. The vertebral bodies may become biconcave in shape, or compression fractures may be apparent. In tubular bones, the loss of trabecular bone may cause the metaphyses to appear radiolucent. Pathologic fractures may occur at multiple sites.
In the tubular bones, bone resorption may be distinguished in 3 sites: the endosteal envelope, the intracortical [haversian] envelope, and the periosteal envelope. These changes are best depicted with magnification radiography; they are quantitated with radiogrametry (see the images below).
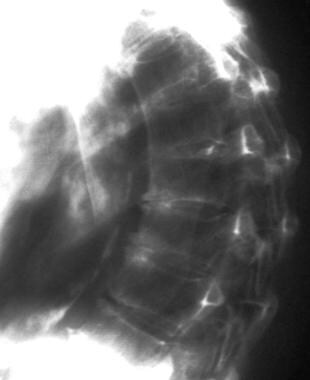
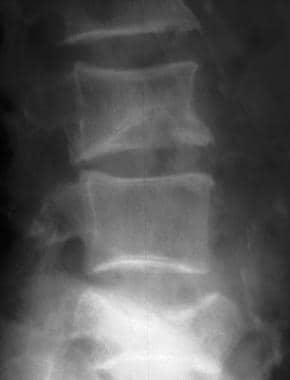
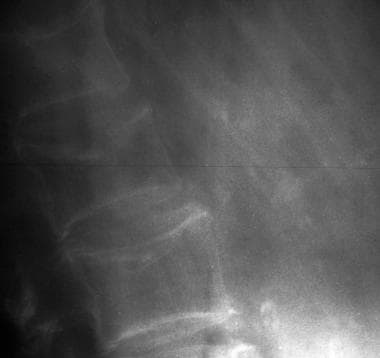
Other radiographic manifestations of osteoporosis include the following:
-
Involvement of the lower dorsal and lumbar spine, proximal humerus, femoral neck, and ribs (These sites are most commonly affected.)
-
Increased radiolucency of bones
-
Decreased number and increased thickness of trabeculae
-
Cortical thinning
-
Juxta-articular osteopenia with trabecular prominence
-
Bone bars (reinforcement lines)
-
Insufficiency fractures
-
Vertebral wedge fractures, fish vertebrae, Schmorl nodes, and decreased heights of vertebrae and accentuation of the cortical outlines (also called picture framing)
-
Absence of osteophytes
-
Compression deformities associated with protrusion of intervertebral disks and prominence of end plates
-
Vertical vertebral striations due to marked thinning of the transverse trabeculae with relative prominence of vertical trabeculae.
Singh index
Plain radiographs of trabecular bone show a distinct pattern. Osteoporosis results in characteristic changes in this pattern and distinctive differences in the appearance of healthy bone and osteoporotic bone. The Singh index is used to assess patterns of trabecular loss. Although of historical interest, it is no longer used in the United States. [43]
The Singh index grading system has always been considered too variable for diagnosis or epidemiologic studies. However, advances in image processing techniques have shown its promise as a method that can overcome the limitations of observer grading. Despite the growing body of evidence that such techniques may be useful, results so far are inconclusive; they may yet provide a tool for the study of osteoporosis. [43]
Radiographic absorptiometry
Absorptiometry is a semiquantitative method of determining BMD. Radiographic absorptiometry is used for peripheral body sites with little overlying soft tissue, such as the hand. The extremity is radiographed simultaneously with an aluminum step wedge, and a densitometer is subsequently used to compare the density of the bones with that of the step wedge. Computer-assisted analysis of paired images obtained at slightly different exposures has yielded more accurate results.
SPA study
Single-photon absorptiometry (SPA) was established for the bone densitometric evaluation of the appendicular skeleton. SPA uses a single-energy source of gamma rays (iodine-125; photon energy, 27.3 keV) or americium-241 (60 keV) to produce a collimated pencil beam, which is tracked across the measurement site. The half-life of 125I is approximately 60 days; its useful life is around 6 months. The transmitted photons are counted by using a sodium iodide crystal/photomultiplier for each point along the track.
Because of the low photon flux and energy source, the technique is usually applied to a peripheral skeletal site, such as the forearm and, less commonly, the heel. The forearm chosen is that of the nondependent arm. To allow correction for soft tissues, the forearm must be placed in a water bath. The mean photon count through the water bath without the interposed limb is used as a baseline value. A reduction in the photon count below this baseline is assumed to be due to the bone. Muscles of the forearm have attenuation effect similar to that of water. The effects of a varying muscle mass are thus eliminated by the water bath.
Although both SPA and DPA were widely used, and although they provide valuable research data, the radionuclide source is a disadvantage. The energy source is subject to decay and must be replaced regularly. The low photon flux can cause the scanning times to be long (up to 40 min), and spatial resolution tends to be poor. SPA machines repeatedly scanned in a single line and were limited (because of the physics of their operating principle) to measuring bone sites that could be either immersed in water or embedded in material with absorption properties equivalent to soft tissue (to simulate homogenous overlying soft tissues).
DPA study
Dual-photon absorptiometry (DPA) is an extension of the SPA principle that was developed to compensate for errors in SPA bone-mass measurements due to the varying composition and thickness of surrounding soft tissues. This deficiency of SPA was overcome by using 2 distinct photon energies, usually gadolinium-153. Photons of different energy are differentially attenuated by bone and soft tissues. Therefore, their absorption by bone, and hence bone density, can be calculated by measuring the percentage of each transmitted beam and then by applying simple simultaneous equations. The source of photons is 153Ga, which emits photons of 2 discrete energies (44 and 100 keV). The scanning approach is similar to that of SPA.
DPA represents an improvement over SPA in that it allows the direct measurement of vertebral or femoral bone density. DPA eliminates the requirement that soft tissue thickness be constant across the scanning path (allowing its use in areas such as the spine and femur). DPA can be used to quantify changes in patients with metabolic bone disease or in those undergoing treatment with drugs that alter bone mineral content.
The desirable characteristics of DPA include its capability in assessing vertebral, proximal femoral, or total body bone content; its independence from effects of marrow fat and other soft tissue; and its relatively low radiation dose. However, it is more expensive than other techniques, it has a longer scanning time, and it is not as widely available as SPA.
SXA study
Single x-ray absorptiometry (SXA) is the x-ray–based equivalent of SPA and uses a filtered x-ray spectrum (55 KeV, 300 µA) with k-edge filtration and solid-state detectors. As with SPA, the arm to be measured must be placed in a water bath to allow correction for the overlying soft tissues. The source and counter move together over the body part being examined, creating an image.
SXA has been used only with the radius and calcaneus. The area of interest is positioned in tissue-equivalent material to produce uniform soft-tissue uptake that can then be subtracted from the image for the calculation of bone density. The distal radius is the most sensitive region for measuring bone density in most disease processes because this site reflects the high turnover of trabecular bone.
The difference in photon absorption between bone and soft tissue allows the calculation of the total bone mineral content in the scanning path. Bone mineral content is expressed as grams of bone mineral per square centimeter imaged.
The equipment is relatively compact and mobile, and scanning usually takes about 5 minutes with the forearm in a standard position. The accuracy is 3%, and the precision is better than 1% in the distal forearm. The radiation dose is less than 0.1 µSv. The Osteoanalyser measures BMD in the heel; scanning is completed in 2 minutes with a precision better than 1%. [44]
Rectilinear scanning is performed in the distal (87% cortical bone) and ultradistal forearm (65% trabecular bone). Results are expressed as BMD or as bone mineral content in grams per square centimeter.
DEXA study
Dual-energy x-ray absorptiometry (DEXA) is very much like DPA except that the radionuclide source is replaced by an x-ray source. The spectrum is heavily filtered with different filters, giving a spectrum with 2 narrow distributions of photons that simulate the spectrum from the radionuclide source. This technique eliminates the need to constantly subtract the soft-tissue thickness as in single-photon measurements; therefore, DEXA permits measurement of the spine and hip.
A deficiency of this method, in the conventional AP projection of the spine, is that the posterior elements, which consist of cortical bone, are included in the result. The mechanical strength of the vertebrae is mainly dependent on the amount of trabecular bone in the vertebral body. Despite this drawback, the low radiation dose, speed of examination, and low cost have made it popular as a clinical screen for osteoporosis.
DEXA overcomes many of the problems of DPA in that it is inexpensive and has high accuracy, precision, and resolution. DEXA has a number of advantages over DPA, including a precision of 1% or less (vs 2-5%), a radiation dose of less than 2 mrem (vs 10-20 mrem), and an examination time of less than 5 minutes (vs 20-30 min). Because of its precision, DEXA is well suited to making serial measurements to monitor the effect of treatment. At present, DEXA is the most precise method for measuring BMD.
False positives and negatives
Conventional radiography is insensitive for diagnosing osteoporosis. At least 30% of the bone mass must be lost before it is recognized.
The precision error (coefficient of variation) is 1% for SPA. The precision error is affected not only by the measurement of technique but also by patient characteristics. Precision error tends to increase in an elderly or osteoporotic population due to factors such as greater difficulty in repositioning and lower mean BMD.
SXA cannot separate trabecular and cortical bone components. The precision of this method is around 1-2%, and the accuracy is ±2-4%.
With DPA, the error of precision and accuracy is 2-3%. The precision of DEXA is 2-6%, and the accuracy is about ±5%. One unavoidable source of error in the dual-photon technique is the distribution of fat in the path of the radiation beam. It is possible to correct for an evenly distributed fat layer across the scanning path, but an uneven distribution introduces error into the measurements. DEXA has some limitations, including artifacts such as degenerative disk disease and osteophytosis in the older spine that can cause a false elevation of BMD.
Computed Tomography
Bone-mineral density (BMD) measurements with a CT scanner have the major advantage that the trabecular component can be identified, and thus the measurements can be confined to these parts. For both single- and dual-energy CT methods, careful calibration of the CT unit must be undertaken. In addition, decalcification and fat replacement of the trabeculae not only affect the BMD but also affect the atomic composition of the area. BMD can thus be measured by outlining the trabecular part of the bone being investigated, by calculating the mean Hounsfield number in the area, and by applying the calibration equation to this measured value. For dual-energy CT, the measurement involves 2 scans at different kilovolt peaks; the measured Hounsfield numbers are applied to a more complicated expression. [14, 9, 18, 19]
(See the image below.)
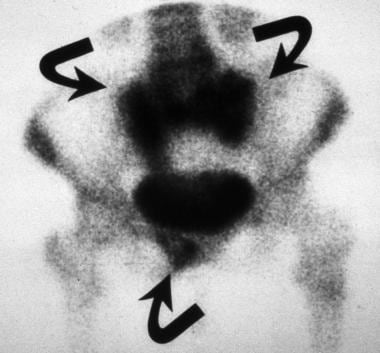
Another promising method involves measurement of the amount of radiation from a monoenergetic gamma-ray source that is coherently and incoherently (Compton) scattered by the bone tissue. Because the amount that is coherently scattered is dependent on Z3 (where Z is the atomic number) and because the amount that is incoherently scattered is dependent on Z, the ratio of coherent to incoherent scatter is sensitive to BMD. By using a well-defined collimation of radiation source and detector, the volume investigated can be well defined and positioned in the trabecular part of the bone being investigated. [45]
The advantage of the CT methods is that the result is a true BMD (in milligrams of hydroxyapatite per unit volume) and that it is measured only in the bone tissue of interest (trabecular bone). The precision of the CT methods is high: 1-2% for the single-energy method and 3-5% for the dual-energy method. The accuracy is also high: 4–7% for the single-energy method, and 3–5% for the dual-energy method.
QCT is generally used to measure bone density in the lumbar spine, though it can be applied to other parts of the skeleton, such as the forearm. The accuracy and scanning time depend on the type of CT scanner used. This technique is the only BMD-measurement method that provides a true volumetric measurement of bone density (in milligrams per cubic centimeters) and a separate measurement of trabecular and cortical bone density. [19]
QCT has been used to assess vertebral fracture risk. It has been found to be superior to other methods for assessing age-related bone loss, for distinguishing fractures, and for diagnostic classification.
Developments in CT technology allow 3-dimensional (3D) volumetric BMD analysis of the proximal femur; high-resolution CT (HRCT) allows the analysis of trabecular structure. QCT bone-density measurements of the lumbar spine can be performed on standard CT scanners with provision of specialized software, and peripheral QCT (pQCT) measurements can be obtained on specially designed small-bore CT scanners.
The measurements are accurate and precise and require a comparatively low radiation dose as compared to that needed for a standard diagnostic CT procedure. QCT is more accurate than DXA in measuring BMD, especially in the spine in the older population group, as CT avoids the effects of degenerative disease and extraneous calcification. Developments in 3D QCT allow assessment of the hip and complicated situations in the spine, as when both scoliosis and vertebral fractures are present.
One major disadvantage of QCT is that artifacts hamper the CT data, reducing its accuracy. The usual sources of error include beam hardening, detected scatter, and system drift. The accuracy of QCT readings can be improved with careful attention to detail. Patients should be well centered and scanned using consistent settings. Reference phantoms can be scanned and the results used to correct for deterministic errors. Another limitation of QCT is a significantly higher radiation dose than that of DEXA. For most clinical purposes, DEXA has remained the method of choice over QCT.
The presence of excess fat in the marrow in trabecular bone in the aging population introduces an error in the BMD measurement of 7-15% per 10% of fat. This problem can be resolved by using dual energy, but at the expense of doubling the radiation exposure to the patient. The accuracy error and precision of QCT are 5-8%.
Magnetic Resonance Imaging
Bone-mineral density (BMD) is the most important factor contributing to bone strength and the risk of fracture. However, studies have shown that changes in bone quality and structure, which influence both bone strength and individual risk of fracture, are independent of BMD. The influence of these other factors is thought to at least partially account for the observed overlap in bone-mineral measurements in patients with, and in those without, osteoporotic fractures, irrespective of measurement site or technique. Therefore, devising a method to assess bone quality and to quantify the trabecular bone structure may be what is important in assessing fracture risk. See also the Fracture Index WITH known Bone Mineral Density (BMD) calculator.
MRI is not yet in the mainstream use in the diagnosis of osteoporosis and is unlikely to become so because of its expense and the time required to obtain a scan. Even so, several noninvasive MR techniques have been shown to provide microstructural information about bone beyond simple bone densitometry. Newitt described a method for characterizing trabecular bone structure on high-resolution MRIs. [46]
Advances in MRI allow spatial resolutions of 80-150 µm and a section thickness of 300-700 µm, allowing resolution of the trabecular structure. Majumdar and Genant used MRI to quantify trabecular bone structure and bone density, both in vivo and in vitro. [47] They used both modified spin-echo and gradient-echo sequences to obtain the images, despite the fact that the technical parameters and the sequence-specific mechanisms affected the depiction of trabecular bone. They concluded that in conjunction with 3D image processing and an understanding of the mechanisms of image formation, these high-resolution images might be used to quantify trabecular bone architecture.
In addition to standard stereologic measures, other parameters may be derived from such images: trabecular bone volume; mean trabecular width; mean trabecular spacing; mean intercept length as a function of angle; parameters such as 3D connectivity, as measured by the Euler number; the fabric tensor in 3 dimensions; and texture-related parameters, such as fractals.
MRI is valuable in the assessment of vertebral body fractures, nonspinal insufficiency fractures, bone mass and strength, and bone marrow edema. The signal-intensity characteristics of bone marrow may allow the differentiation of neoplastic fractures from accompanying osteoporosis.
Because the features of osteoporotic fractures and fractures due to other infiltrative processes overlap, false-positive and false-negative results are possible.
Ultrasonography
Langton first described the measurement of broadband ultrasound attenuation (BUA) in the calcaneus as a potential indicator of hip fracture risk. [48] The concept is based on the knowledge that the speed of sound and attenuation of sound wave are affected by the density, compressibility, viscosity, elasticity, and structure of the material it is traveling through. This technique marked a departure from the conventional methods of bone densitometry that used ionizing radiation. Ionizing radiation is attenuated at the atomic level, whereas ultrasound is attenuated at the macroscopic structural level. Some therefore suggest that BUA depends on the macroscopic structure of cancellous bone in addition to the bone-mineral density (BMD) assessed by using the ionizing radiation techniques. [24, 49, 16, 17]
BUA measurement in the calcaneus requires 1 transducer with 2 broadband ultrasound transducer components: One acts as the transmitter, and the other acts as the receiver. For a given material, ultrasound attenuation is always the same; this is known as the BUA index. To determine the attenuation index of any material (including bone), a broadband of ultrasound frequencies is passed through the full thickness of the material. The amplitude spectrum of the received signal is then compared with the spectrum of a reference material (water). By recording the frequency spectrum through water with and without the heel in position, a plot of attenuation with frequency is achieved. The difference between the 2 spectra is then plotted against frequency, giving a straight-line graph, the slope of which is the BUA index (in decibels per megahertz). The ultrasound frequencies used are in the range of 0.1-1 MHz. This range has become known as BUA.
The relationship between the index and BMD is not straightforward, however. The BUA index is highly influenced by bone structure, not only with regard to the number and thickness of bone trabeculae but also regarding their orientation with respect to the ultrasound beam. A plethora of quantitative ultrasonography (QUS) devices are now available. [12]
QUS for bone analysis is a nonionizing method in which the calcaneus is the measurement site. This technique is both cost-effective and accurate for identifying patients at risk for osteoporotic fracture. QUS has been scientifically validated in both fundamental in vitro studies and clinical in vivo studies. Clinical studies have shown that QUS parameters are sensitive to age-related changes, that they may be useful in distinguishing osteoporotic patients, and that they offer a prospective prediction of fracture risk comparable to that of axial DXA.
Normative data have been defined for several devices. QUS is more diverse than conventional bone densitometry, and both cortical and cancellous bone may be assessed to note their dissimilar pathophysiologic behavior. The 2 fundamental parameters of attenuation and velocity are often device specific and implemented or combined into proprietary parameters.
Comparisons of BMD obtained using BUA methods with BMD using more established techniques have resulted in relatively poor correlation, with r values ranging from 0.36 (BUA vs single-photon absorptiometry [SPA] or quantitative CT [QCT]) in osteoporotic patients to 0.8 (BUA vs SPA) in rheumatoid patients. The poor correlations may be partly attributed to the different sites and to the different physical quantities measured using the 2 techniques.
Whether QUS can be used to monitor treatment has not been conclusively shown. In monitoring the response to treatment, QUS can reliably show differences in responses between individuals. These differences can be predictive of long-term differences in bone mass that are not simply due to measurement error.
A number of factors can affect the accuracy and precision of BUA and produce false-positive or false-negative results. The anatomically incorrect placement of the region to be examined is one of these factors. Other factors are patient specific and may affect bone measurements; these are variability in bone width and soft-tissue thickness or composition; marrow composition; and temperature. Error in measurement can be introduced by diffraction, which affects both attenuation and velocity measurements and is device specific.
Nuclear Imaging
Other than single-photon absorptiometry (SPA) and dual-photon absorptiometry (DPA), as discussed above, nuclear medicine is not used to measure bone-mineral density (BMD). However, bone scans may be useful in diagnosing insufficiency fractures that are not visible on plain radiographs. [50]
Schmitz and associates used fluorodeoxyglucose (FDG) positron emission tomography (PET) in an attempt to differentiate patients with osteoporosis or preclinical osteoporosis from those with other pathologic fractures. [51] Results indicated that acute vertebral fractures originating from osteoporosis or preclinical osteoporosis tend to have no pathologically increased FDG uptake. Because high FDG uptake is characteristic of malignant and inflammatory processes, FDG-PET may be potentially useful for differentiating osteoporotic vertebral fractures and pathologic ones.
Radionuclide bone scans are particularly useful for screening the whole skeleton for abnormal activity at a site of osteoporotic fractures. The activity pattern is usually different in bony metastases. [52] Radionuclide scans are particularly helpful in the diagnosis of sacral insufficiency fractures, for which the appearances may be characteristic.
Although isotopic bone scans have high sensitivity, their specificity is low because areas of increased uptake may be seen at the sites of fractures, infection, metabolic bone disease, and metastases. The sensitivity of isotopic bone scans in the detection of osteoporotic fractures drastically decreases in elderly patients with osteoporotic fractures and in patients taking steroids.
Questions & Answers
Overview
How is bone mineral density (BMD) measured in patients with osteoporosis?
What are the guidelines for screening and monitoring osteoporosis?
How are vertebral fractures treated in patients with osteoporosis?
What are the limitations of imaging techniques in the diagnosis of osteoporosis?
What is the role of conventional radiography in the workup of osteoporosis?
What is the role of the Singh index in the diagnosis of osteoporosis?
What is the role of absorptiometry in the workup of osteoporosis?
What is the role of single-photon absorptiometry (SPA) in the workup of osteoporosis?
What is the role of dual-photon absorptiometry (DPA) in the workup of osteoporosis?
What is the role of single x-ray absorptiometry (SXA) in the workup of osteoporosis?
What is the role of dual-energy x-ray absorptiometry (DEXA) in the workup of osteoporosis?
What is the accuracy of radiography for the diagnosis of osteoporosis?
What is the role of CT scanning in the workup of osteoporosis?
What is the role of MRI in the workup of osteoporosis?
What is the role of ultrasonography in the workup of osteoporosis?
What is the role of nuclear imaging in the workup of osteoporosis?
-
Involutional osteoporosis. T and Z scores in an 80-year-old woman.
-
Involutional osteoporosis. T and Z scores in an 80-year-old woman.
-
Involutional osteoporosis. T and Z scores in an 80-year-old woman.
-
Involutional osteoporosis. T and Z scores in an 80-year-old woman.
-
Involutional osteoporosis. Note the overall reduction in the bone density and moderate kyphosis of the dorsal spine.
-
Involutional osteoporosis. Note the lateral wedge fracture of L3 and a central burst fracture of L5. The patient had a recent fall.
-
Involutional osteoporosis. Note the considerable reduction in the overall bone density and lateral wedge fracture of L2.
-
Involutional osteoporosis. Insufficiency fractures of the sacrum and the pubic rami are seen on an isotopic bone scan as a characteristic H, or Honda, sign (arrows), which appears as intense radiopharmaceutical uptake at the fracture sites.