Overview
The care of children with cancer has made remarkable progress over the last 5 decades, with the cure rate now approaching 80%. Since the first large cohort of patients was cured in the 1970s, much has been learned about the long-term effects of exposure to chemotherapy, radiation, and surgery in childhood. Studies have shown a mortality rate of 18% within 30 years for 5-year survivors. [1] Additionally, over 60% of childhood cancer survivors self-report having a chronic illness and 98% have identifiable abnormalities in end-organ function. [2, 3] Moreover, many survivors face psychological or societal barriers, such as post-traumatic stress disorder (PTSD) and difficulties obtaining insurance. [4, 5] The late effects of exposure to chemotherapy, radiation and surgery make the care of survivors highly complex and mandate structured, coordinated long-term follow-up.
Likewise, many children are now surviving hematopoietic stem-cell transplantation (HSCT) (see related histology slide below) and require structured long-term follow-up care. The number of long-term survivors of childhood HSCT will continue to increase as new indications for transplantation emerge, as donor pools expand (eg, umbilical-cord blood, haploidentical donors), ex vivo expansion of hematopoietic stem cells becomes feasible, [6] and as supportive care improves. In addition to complications seen from exposure to chemotherapy and radiation, patients undergoing allogeneic transplantation can experience unique late effects secondary to graft versus host disease (GVHD) and autoimmunity.
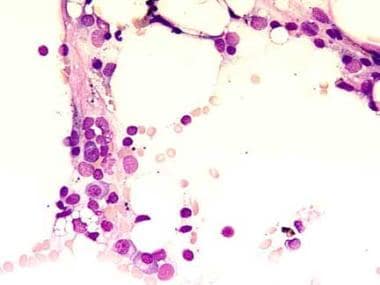
Many of the special concerns facing long-term survivors have been highlighted in studies from Europe and the United States. In a study of the European Bone Marrow Transplant Registry, [7] of 798 patients who survived more than 5 years after transplantation, 328 were children. Although most were apparently well, survivors at 10 years and 15 years after HSCT continued to have an increased risk of death compared with a group of individuals from the healthy population who were age-matched and sex-matched. Causes of death were related to relapse of the underlying cancer, development of a second cancer, chronic GVHD, or transfusion-acquired viral infection. Certain patients were at higher risk for late morbidity and mortality than others; high-risk patients included male subjects, recipients of stem cells from female donors, patients younger than 10 years, those with nonidentical donors, and those who received radiation therapy in the transplant preparative regimen.
In a study from the United States, long-term survivors of pediatric bone marrow transplantation followed in the Bone Marrow Transplant Survivors Study were compared with survivors of childhood cancer treated without bone marrow transplant from the Childhood Cancer Survivor Study. [2, 8] Survivors of bone marrow transplantation were more likely to have a severe or life threatening condition (relative risk [RR] = 3.9), more than one chronic condition (RR = 2.6), functional impairment (RR=3.5), and activity limitations (RR = 5.8) than conventionally treated patients. These data reinforce the need for marked vigilance in ensuring proper screening and management of long-term survivors of bone marrow transplantation.
While screening and preventative guidelines for survivors of HSCT have been developed, [9, 10, 11] these guidelines have been driven primarily by expert opinion with limited pediatric data. To stimulate and facilitate further research, the NCI and NHLBI held the First International Consensus Conference on Late Effects after Pediatric Hematopoietic Cell Transplantation on April 28 and 29, 2011. Out of this conference, a series of 6 papers were published on topics considered to be the most critical for future research efforts. These papers covered topics that included (1) methodological challenges [5, 12] ; (2) immune reconstitution and tolerance [13] ; (3) organ toxicities and metabolism [14] ; (4) quality of life, functional outcomes, and neurocognitive outcomes [15] ; (5) endocrine challenges [16] ; and (6) consensus guidelines for long-term follow-up after pediatric HCT. [17]
This review highlights some of the special needs of children who survive after HSCT. The clinical manifestations of late complications are discussed to help physicians recognize them early. Specific screening and prevention measures are provided. This chapter is divided into discussions of (1) immune deficiency and chronic GVHD after HSCT; (2) secondary malignancies after HSCT; and (3) physical complications after HSCT.
Immunosuppression and Graft Versus Host Disease After Hematopoietic Stem Cell Transplantation
Immune suppression after transplantation is a major barrier to success, with serious infections accounting for 4-20% of late deaths. [18] Preparative regimens, graft versus host disease (GVHD) prophylaxis, and GVHD itself contribute to immunosuppression. The speed with which immune function recovers after hematopoietic stem cell transplantation (HSCT) depends on many factors, including the type of graft (eg, allogenic, autologous, syngenic, T-cell replete, T-cell depleted), the presence or absence of acute or chronic GVHD, the conditioning regimen, the type of postgrafting immunosuppression, and the underlying disease for which HSCT was undertaken.
Although innate immunity, including cytotoxic and phagocytic function, usually recovers by approximately 100 days after non–T-cell–depleted transplantation, adaptive immunity (CD4(+) T cells), often takes 1 full year or longer for recovery. Full reconstitution is completed within 1-2 years, although chronic GVHD and its treatment can cause a delay. [19] Given this sequence of immune recovery, patients are at highest risk for severe bacterial and fungal infections early after transplant, with lessening risk as innate immunity recovers. However, without functional adaptive immunity, patients remain at risk for severe viral infections as well as bacterial and fungal infections during the first year after transplant and sometimes longer.
Adaptive immune dysfunction, either from the conditioning or chronic GVHD, results in severe hypogammaglobulinemia, adding to the risk of infections. Treatment with immunoglobulin can reduce the frequency of infections in patients with severe hypogammaglobulinemia. [20]
A study examining the kinetics of numerical T-cell recovery found that CD4+ and CD8+ cells can show signs of recovery as fast as 3 months after transplantation, although CD8+ recovery may be delayed as long as 8 months after umbilical cord blood transplantation. [21] In terms of function, an older, prospective study of 64 children undergoing transplantation with T lymphocyte–depleted marrow from matched family donors or matched unrelated donors showed that T-lymphocyte response to T-cell alloantigen normalized 9 months after transplant. [22] However, the response to nonspecific antigen (eg, phytohemagglutinin, concanavalin A) stayed impaired until 12 months after transplantation, and the response to specific antigen (eg, tetanus toxoid, Candida albicans antigen) was delayed and did not normalize until 36 months after transplant.
When to optimally reimmunize patients post transplantation remains an open research question. An international committee developed a unified set of vaccine guidelines in 2009. [23] While this is helpful, immunization guidelines change rapidly, and, as more knowledge is gained about the kinetics of immune reconstitution, it is likely that personalized revaccination timetables will be developed for each patient based on donor, stem cell source, age, and other factors.
Graft versus host disease
GVHD can be divided in 2 classes, depending on when it occurs after transplantation. Acute GVHD is defined as GVHD that occurs within 100 days after transplantation, and chronic GVHD is GVHD that occurs after 100 days. In discussing the late effects of HSCT, most are related to chronic GVHD, which is discussed below.
Certain patients, including those who received transplants from human leukocyte antigen (HLA)-nonidentical donors, those with previous acute GVHD, and those older than 20 years at the time of transplantation are more predisposed to develop chronic GVHD. [24, 25] Predictive factors for the development of chronic GVHD include the degree of HLA-match, the type of GVHD prophylaxis administered, the degree of acute GVHD, ongoing chronic viral infections, and the stem cell source. A meta-analysis from 9 randomized trials of 1111 patients demonstrated that recipients of peripheral blood stem cell transplants (PBSCT) were at increased risk for chronic GVHD. [26] The overall incidence of chronic GVHD among patients who underwent PBSCT was 62% compared with 52% among those who underwent bone marrow transplantation (BMT; odds ratio [OR] = 1.92; 95% confidence interval [CI], 1.47-2.49; P< 0.001).
Negative prognostic factors in patients who have developed chronic GVHD include a donor positive for cytomegalovirus (CMV), acute GVHD after transplantation, and nonlymphomatous underlying disease for which the transplantation was undertaken. Also, GVHD duration of longer than 3 years, a platelet count of < 100 X9/L (< 100 X3/mcL), and a history of acute hepatic GVHD have been associated with poorer survival. [27]
Many organs and systems can be involved in chronic GVHD, especially the skin (scleroderma), liver (cholestasis and cirrhosis), eyes (sicca syndrome or cataracts), mouth (mucosal thickening), lungs (fibrosis), GI tract, and neuromuscular system. Biopsy of skin involvement shows markedly decreased numbers of Langerhans cells, deposition of lymphocytes and immunoglobin complexes, and often hyperkeratosis. Hepatic involvement is less common in chronic GVHD than in acute GVHD and usually reflects cholestatic abnormalities, with hyperbilirubinemia and, in rare cases, cirrhosis. Keratoconjunctivitis or sicca syndrome presents with ocular burning, irritation, decreased tear production, and photophobia. Keratoconjunctivitis sicca can be diagnosed by performing the Schirmer test for tear production.
Chronic GVHD can also resemble Sjögren syndrome because of the presence of xerostomia, xerophthalmia, and enlargement of the salivary glands. Laboratory evidence of autoantibodies, (ie, anti-Ro [SS-A] and anti-La [SS-B]), along with high percentage of CD8 lymphocytes compared with CD4 lymphocytes, help to differentiate these 2 entities.
A particularly severe pulmonary complication associated with chronic GVHD is late-onset, noninfectious pulmonary complications (LONIPC). This represents a group of pulmonary disorders, including bronchiolitis obliterans, bronchiolitis obliterans with organizing pneumonia, and idiopathic pulmonary syndrome. A study examining 97 children who survived allogeneic HSCT reported the incidence rate of LONIPC at 10%, consistent with the incidence reported in the adult literature. [28] Multivariate analysis revealed high-risk underlying disease and extensive chronic GVHD as significant risk factors for LONIPC.
Although GI involvement is less common in chronic GVHD than in acute GVHD, it can result in severe dysphagia, pain, and weight loss. A study examining 17 children with chronic GVHD of the intestine revealed a dismal outcome, with 24% of the patients dying or requiring total parenteral alimentation. [29] Multiorgan chronic GVHD is associated with more weight loss than GVHD confined to one organ and often results in growth retardation. [30]
In rare instances, nephrotic syndrome, bullous esophagitis, and unexplained effusions can occur. Also, patients who have chronic GVHD, especially those receiving immunosuppressive therapy, are at increased risk for Pneumocystis carinii pneumonia (PCP) or Toxoplasma gondii infection and should receive trimethoprim-sulfamethoxazole prophylaxis. Cardiac involvement is distinctly uncommon, although findings consistent with chronic GVHD have been identified in the myocardium of some patients. Complete heart block thought to be secondary to chronic GVHD has been reported in an infant. [31] Hematologically, thrombocytopenia, autoimmune hemolytic anemia (AIHA), or eosinophilia can occur in association with chronic GVHD.
No specific laboratory tests are available to detect, monitor, or follow up chronic GVHD, and definitive diagnosis of GVHD is only possible with histopathologic examination of biopsy material along with clinical correlation. Despite the lack of laboratory correlates, various interventions are under investigation as possible agents or methods to decrease the risk and the severity of GVHD after HSCT. These include both pretransplant and posttransplant interventions and include pharmacologic agents posttransplant to treat chronic GVHD and the use of graft engineering pretransplant (such as with CD34 selection) to reduce the risk of GVHD. [32, 33]
In a preliminary study, Salas et al identified elevated serum prolactin levels as a potential biomarker for chronic GVHD. Long-term survivors of allogeneic hematopoietic cell transplantation who had hyperprolactinemia were 6.4 times more likely to have active chronic GVHD, compared with those who had normal prolactin levels (P< .001). [34]
Although many agents are under investigation, the mainstay of therapy has been, and remains, corticosteroids. Multiple earlier trials comparing steroids alone to combinations of steroids plus cyclophosphamide, mycophenolate mofetil (MMF), procarbazine, anti-thymocyte globulin, and azathioprine did not demonstrate improved outcomes. A randomized trial comparing prednisone with prednisone plus cyclosporine showed reduced toxicity from the corticosteroids but without significant improvement in survival. [35] A randomized, placebo-controlled trial that examined the addition of MMF to conventional therapy did not show any benefit from the addition of MMF. [36]
A study of 188 adult and pediatric transplant programs examining management practices for chronic GVHD through self-reported surveys found that 28% felt they had great success in treating patients with systemic corticosteroids, and 13% had similar success with cyclosporine or mycophenolate mofetil; less success and experience were reported with other agents. [37]
Despite the lack of efficacy of agents tried to date, novel approaches are under investigation. Two intriguing approaches to the treatment of chronic GVHD involve the infusion of mesenchymal stem cells and the use of pentostatin. In particular, a phase II trial of pentostatin had a 55% response rate. [38] Although the data are immature and mostly in case reports, series, or early phase trials, these therapeutic options warrant further investigation. [39]
Findings by a phase II study by Gao et al suggested that the repeated infusion of mesenchymal stromal cells might inhibit chronic graft-versus-host disease symptoms in patients after HLA-haploidentical hematopoietic stem-cell transplantation. Further studies are needed to examine these results. [40]
Another approach is photopheresis, in which lymphocytes are treated with psoralen and UV light. Although the data in children with chronic GVHD are mostly anecdotal or retrospective, in one study of 54 children with chronic GVHD, photopheresis had a 75% overall response rate; however, the peak response did not occur until 9 months into therapy. [41] For those with skin involvement of chronic GVHD, some early investigations have suggested a possible role for psoralen and UV light therapy or topical tacrolimus. [42, 43]
In summary, a diagnosis of chronic GVHD should be suspected in any patient who received allogeneic HSCT and presents with sclerodermatous changes, dry eyes or prolonged conjunctivitis, thickened oral mucosa, or new-onset organ damage.
Secondary Malignancies After Hematopoietic Stem Cell Transplantation
Secondary malignancy is a risk after radiation alone or in combination with chemotherapy and is the second leading cause of death in children treated for malignancies. [1] These risks also apply to patients who undergo hematopoietic stem cell transplantation (HSCT).
A study examining second malignancies in 3,182 children who underwent allogeneic HSCT for leukemia revealed probabilities of new, invasive solid tumors to be 0.9%, 4.3%, and 11% at 5 years, 10 years, and 15 years posttransplant, respectively. [44] Risk was significantly elevated for developing solid tumors of the tongue, salivary glands, brain, thyroid bone and connective tissue, and malignant melanoma. Another study found a markedly increased incidence of squamous cell carcinoma and basal cell carcinoma post HSCT, with 20-year cumulative incidences of 6.5% and 3.4%, respectively. [45] A report on 1487 children who underwent autologous hematopoietic stem cell transplantation for malignancies found an overall cumulative incidence of second malignancies at 10 years of 2.6%. This was a 24-fold increase in risk compared with the age- and sex-matched general population. [46]
The increased risk of malignancies following HSCT has been a consistently reported phenomenon. [47, 48]
The above cited study of children with leukemia found that although the risk of solid tumors increased with time, the risk of posttransplant lymphoproliferative disorder (PTLD), which is a form of lymphoma associated with Epstein-Barr virus, was highest during the first 5 years after transplant. [44] The PTLD incidence of 1% at 5 years was consistent with previously published results. PTLD results from T-lymphocyte dysfunction after transplant secondary to immunosuppression or depletion. This allows EBV-infected B lymphocytes to proliferate in uncontrolled fashion, resulting in lymphomatous transformation; 90% of PTLD cases are EBV positive. [49, 50] T-cell PTLD is rare, with only a few cases reported in the literature. [51] The incidence of PTLD is substantially higher after solid-organ transplant than after HSCT. PTLD is often fatal (uniformly in the study cited above) and occurs with the highest frequency in patients who receive T-cell–depleted bone-marrow grafts because these patients tend to have greater immune deficiency for longer periods of time. T-cell depleted grafts generally reduce the risk of acute graft versus host disease (GVHD), although they increase the risk of PTLD. [52]
Physical Complications After Bone Marrow Transplantation
Survivors of hematopoietic stem cell transplantation (HSCT) in childhood often have long-term organ damage after transplantation from their exposure to high-dose chemotherapy and radiation. This section reviews many of the organ systems that may be affected by the conditioning for HSCT. Growth failure, gonadal dysfunction and thyroid dysfunction are the most common endocrine sequelae of HSCT and can seriously disrupt the patient's quality of life.
Growth failure
Growth failure is a complication of HSCT unique to children, and growth hormone deficiency is a common endocrinopathy among childhood cancer survivors. It is seen most often following radiation to the hypothalamic-pituitary axis. [53]
Radiation used as part of conditioning for HSCT damages the neuroendocrine organs required for growth hormone production, as well the bones and cartilage themselves. [54] Radiation is the most important factor that causes growth failure in children undergoing HSCT. A study of 181 patients who underwent HSCT as children revealed that 80% achieved an adult height within the normal range for a healthy population. Among those who did not achieve normal height, radiation was the leading cause of growth failure. [55] Of note, younger age and male gender were risk factors for more severe loss of height. A separate study of 23 children with acute myeloid leukemia (AML) conditioned with chemotherapy alone demonstrated no growth impairment, supporting the importance of radiation in the pathology of posttransplant growth failure. [56]
Fractionation of the radiation dose improved final height, [55] although hyperfractionation did not provide any further improvement. [57] Although some studies support damage to the spinal column as a cause of growth failure (as determined by a reduced sitting height, rather than standing height), [54, 57] others hold that growth hormone deficiency is the primary culprit. The onset of growth hormone deficiency depends on the age of the child at the time of irradiation, the dose of radiation used, and the time elapsed from the radiation. [58] An exposure of 30 Gy seems to represent the dose at which the risk of growth hormone deficiency markedly increases. [59] A study of 90 children with growth hormone deficiency showed that growth hormone replacement in patients who were younger at transplant benefited from growth hormone replacement. Those who were older than 10 years at the time of transplant did not experience significant improvement in final height. [60]
Despite the potential benefits of growth hormone replacement, some hesitancy surrounds its use in childhood cancer survivors based on the increased risk of a second malignancy reported in several small cohorts of patients. [61, 62, 63, 64] In the initial publication reporting a potential increase of second malignancies in childhood cancer survivors treated with growth hormone, 361 childhood cancer survivors who were treated with growth hormone were compared with those who were not treated with growth hormone. No increased risk of recurrence of the original cancer was noted; however, an apparent increase was observed in the risk of developing a second, unrelated solid tumor.
Although this study did raise a potential concern, the number of second malignancies was small (20 of 361 survivors treated with growth hormone), the relative risk for developing a second malignancy was 2.1 and seemed to decrease over time. All of the second malignancies were solid tumors, and 9 of them were meningiomas. [62]
Overall, the risk of developing a second malignancy from growth hormone therapy remains unclear and should be weighed against the benefits in childhood cancer survivors.
Gonadal dysfunction
High-dose alkylator and radiation therapy used as conditioning for HSCT invariably affect ovarian and testicular function. When discussing gonadal dysfunction, 2 distinct aspects must be considered: hormone production and fertility. Although physiology links these gonadal functions in women, they often remain distinct in terms of effects of therapy in men.
The ovaries contain approximately 2 million primordial follicles at birth, with no capacity to increase that reserve. Losing follicles primarily through atresia, the normal human ovary has 300,000 primordial follicles left at menarche. When only approximately 1000 remain, menopause ensues (at 50.4 y in the Western world). [65, 66, 67] At the moment of radiation or high-dose alkylator exposure, the number of remaining follicles is reduced. If, after exposure, less than 1000 follicles remains, then acute ovarian failure occurs, with clinical features of menopause. If more that 1000 remain, normal menses may resume, although when the ovarian reserve will diminish to 1000 follicles with resultant menopause is difficult to predict. This results in premature menopause.
Because the number of remaining follicles decrease as a function of age, the dose of radiation required to induce acute ovarian failure decreases with age. A dose of 14 Gy, a typical dose for total body irradiation (TBI), induces acute ovarian failure in a 30-year-old woman. [68] A similar dose given to a 10.5-year-old female likely results in premature menopause by age 13 years.
Alkylators add to the effect of radiation. One study showed that 9 of 16 (56%) prepubertal females (mean age of 6.1 y at the time of HSCT) progressed through puberty with a normal onset of menses and normal estrogen levels after undergoing HSCT with high-dose chemotherapy and hyperfractionated TBI. [69] However, the 7 remaining females (mean age, 8.6 y) required hormone replacement therapy for biochemical and hormonal ovarian failure after HSCT.
Several case series have reported the resumption of ovarian function after initial ovarian failure following HSCT. [70] The mechanism remains unclear. Another study of females who received transplantation for sickle cell disease showed that 7 of 10 females conditioned with busulfan and cyclophosphamide without TBI experienced severe ovarian failure that required estrogen replacement. [71] This stresses the importance of both TBI and alkylating agents in the etiology of ovarian failure in females undergoing HSCT.
Ovarian failure or premature menopause often manifests with high levels of luteinizing hormone (LH) and follicle-stimulating hormone (FSH) with or without decreased estrogen levels, although all eventually progress to a low-estrogen state. Measurements of antimullerian hormone (AMH) may be useful in assessing ovarian reserve. [72] Similar to postmenopausal women with low estrogen levels, females who experience acute ovarian failure or premature menopause following HSCT seem to be at risk for early reduced bone mineral density, [73] bone fractures, [74] lipid disorders, and ischemic heart disease. [75, 76, 77] Use of steroids and radiotherapy can potentiate the risk of reduced bone mineral density.
Ovarian failure after HSCT responds well to estrogen replacement. The known risks of estrogen (ie, predisposition to endometrial and breast cancer [78] ) must be weighed against the risks of bone and heart disease. The role of bisphosphonates to help prevent low bone mineral density and to reduce the risk of fractures in patients with low bone mineral density may be promising in patients undergoing HSCT, although further study and randomized trials are needed. [79]
In terms of fertility, female patients who retain their reproductive potential and become pregnant after HSCT have a high risk for pregnancy complications, including premature deliveries (20% in patients vs 6% in the healthy population), low birth weight infants (23% in patients vs 6% in the healthy population), and cesarean delivery (42% in patients vs 16% in the healthy population). [80] However, rates of infant congenital anomalies and malignancies do not appear to be increased in females exposed to chemotherapy, radiation, or both as therapy for childhood cancer. [81, 82]
Conditioning for HSCT in males differentially effects hormonal gonadal function (ie, testosterone production) and fertility (ie, sperm production) Most prepubertal males undergoing HSCT with chemotherapy-only conditioning regimens progress through puberty normally and maintain testosterone levels within the normal range. [83] Nonetheless, male gonadal function can be affected. In one study, hypogonadotropic hypogonadism was observed in 19% of 31 male patients who underwent chemotherapy as part of preparative regimen for HSCT, although all patients recovered normal gonadal function by 3 years follow up. [84]
Male fertility, on the other hand, is frequently profoundly affected by conditioning for HSCT. In a prospective study of 64 male patients undergoing HSCT with various conditioning regimens, the overall rate of azoospermia was 70%. Those who experienced recovery of sperm production included 90% of the patients conditioned with cyclophosphamide alone, 50% of those conditioned with cyclophosphamide and busulfan, and 19% of those conditioned with cyclophosphamide plus TBI. [85] Of those men who retain fertility and have offspring, no increased risk of congenital anomalies among their children is noted. [85, 86]
Thyroid dysfunction
In a study of 791 children who underwent HSCT at a single institution, but with different conditioning regimens and underlying diseases, 30% developed hypothyroidism, with 20% requiring thyroid hormone replacement. [87] Although far less common, 23 patients experienced hyperthyroidism. On multivariate analysis, preparative regimen, patient age, and disease type were the most significant factors associated with thyroid dysfunction. Busulfan and TBI, younger age (< 10 y), and a history of Hodgkin lymphoma were the largest risk factors.
In addition to thyroid dysfunction, survivors of HSCT are at increased risk for secondary thyroid cancers. A large, retrospective study of 70,859 patients found an overall observed to expected ratio of 3.26. Those who were younger than 10 years at the time of HSCT were at particularly high risk, with a relative risk of 24.61, and females had a higher risk that males (relative risk of 2.79). [88]
In addition to a thorough history and physical examination to elicit any signs or symptoms of endocrine dysfunction, all patients who have undergone HSCT and had exposure to alkylators or gonadal radiation should have serum follicle-stimulating hormone (FSH), luteinizing hormone (LH), and estrogen or testosterone tests at entry to long-term follow-up, and any patients who have had exposure to chest or neck radiation should have thyroid function tests upon entry to long-term follow-up. Any abnormalities should prompt a referral to an endocrinologist or reproductive specialist for a thorough assessment.
Pulmonary dysfunction
Relatively few children surviving hematopoietic stem cell transplantation (HSCT) have clinically significant pulmonary symptoms; however, as many as 33-55% have abnormal pulmonary function test (PFT) results and abnormal lung function. [89, 90, 91] In particular, total body irradiation (TBI) may confer a higher risk of late pulmonary complications.
A retrospective review of children who received TBI as part of their conditioning found that 74% had PFT result abnormalities 3 years after transplant. [92] Late pulmonary complications of HSCT in children can be roughly divided into 2 types: late-onset infectious pulmonary complications (LOIPC) and late-onset noninfectious pulmonary complications (LONIPC). In terms of LOIPCs, the risk is modified by the degree of ongoing immune suppression. The greater the immune suppression, the greater the risk of pulmonary infection. Patients receiving immune suppression remain at risk for fungal, bacterial, and viral infections. [93] Although cytomegalovirus (CMV) pneumonitis is a common complication in the immediate peritransplant period and has a high mortality rate, very late development of CMV pneumonitis has also been reported. [94]
LONIPCs include various pathological entities that appear after approximately 100 days from HSCT. These include bronchiolitis obliterans, bronchiolitis obliterans with organizing pneumonia, and idiopathic pneumonia syndrome or interstitial pneumonitis. The incidence of LONIPCs has been reported to be 10-25%, mostly in adult patients. [95, 96] A study of 97 pediatric patients found an incidence rate of 10.3%. Multivariate analysis in that study revealed chronic graft versus host disease (GVHD) and high-risk underlying disease as significant risk factors for LONIPCs. [28] LONIPCs have been associated with a significantly worse mortality. [28, 97] Symptoms can include cough, wheezing, or recurrent infections. Chest radiography and CT scanning may reveal infiltrates. A definitive diagnosis may require bronchoscopy with biopsy. [98]
The pathogenesis of LONIPCs has not been fully elucidated. However, the association with chronic GVHD supports the theory that alloimmune reactions can play a significant role. [99, 100] Consistent with the probable role of alloimmunity, treatment of LONIPCs has been based on immunosuppressive therapy, such as mycophenolate, azathioprine, corticosteroids, and prompt treatment of infections. [101] Newer approaches have involved immune modulators, such as infliximab, for which limited data are available. [102] In a retrospective study of 144 adult patients undergoing HSCT, only 2% of those who received reduced-intensity conditioning developed bronchiolitis obliterans, as opposed to 15% of those following myeloablative conditioning. [103] Findings such as these may suggest that as the increased use of reduced-intensity conditioning may decrease the incidence of LONIPCs.
In addition to a thorough history and physical examination to elicit any pulmonary signs or symptoms, all patients who have undergone HSCT and had exposure to bleomycin or pulmonary radiation should have screening pulmonary function tests upon entry into long-term follow-up. Any abnormalities should prompt a referral to a pulmonologist. Patients should be counseled on ways to maintain lung health, including getting regular exercise and avoiding tobacco smoke.
Cardiac dysfunction
Cardiac toxicity from HSCT can manifest in two main forms: structural (eg, valvular abnormalities or coronary artery disease) or functional (eg, cardiomyopathies). The major risks of long-term cardiotoxicity are primarily related to treatment before HSCT, [104] particularly anthracycline therapy at doses of more than 300 mg/m2, [105, 106, 107] ablative-dose cyclophosphamide of more than 150 mg/kg, chest radiation therapy, and TBI. [108]
In a prospective analysis of 162 children who underwent allogeneic HSCT, the 5-year cumulative incidence of cardiac impairment (reduced shortening fraction) was 26%. TBI and TBI combined with pre-HSCT anthracyclines were significant risk factors for reduced cardiac function in univariate analysis. [91] Another study of 112 children with malignancies who survived HSCT found a 10-year cumulative incidence of cardiovascular complications of 11%. These complications included abnormal echocardiography findings, hypertension, and cerebrovascular injuries. [109] The cumulative risk of developing cardiac dysfunction increases over time, a finding consistent with that seen in late anthracycline toxicity without HSCT. [107, 110] Thus, continuing life-long cardiac screening after exposure to anthracyclines or cardiac radiation is important.
Cardiac dysfunction may not be identified with conventional testing at rest. A study using exercise assessment of cardiac function in children pre-HSCT and post-HSCT found significantly decreased cardiac function during exercise stress echocardiography in 50% of pediatric patients at 7 years after HSCT. [111] Abnormalities included decreased exercise time, decreased maximal oxygen consumption, and decreased ventilatory anaerobic thresholds. Only 10% of patients had abnormalities after echocardiography at rest, and none had abnormalities on ECG alone. The degree of cardiac abnormalities varied with the underlying disease. Therefore, referring any patients with cardiac symptoms for comprehensive cardiac assessment is crucial.
Although not due to direct cardiac damage, hormonal changes as a consequence of gonadal-toxic therapy may predispose patients to heart disease as well. Early menopause has been associated with increased mortality due to ischemic heart disease. [75, 76, 77] Women and girls who develop estrogen deficiency as a consequence of HSCT (as discussed above) lose the normally protective effects of estrogen against coronary artery disease. Radiation to the heart increases the risk of coronary artery disease at a young age, [108] and a relative estrogen deficiency may accelerate that process. Thus, recognizing gonadal dysfunction in women who underwent HSCT at a young age and instituting appropriate hormonal replacement to reduce the risk of coronary artery disease is important.
In addition to a thorough history and physical examination to elicit any cardiac signs or symptoms, all patients who have undergone HSCT and had exposure to anthracyclines or cardiac radiation should have undergo echocardiography and ECG upon entry into long-term follow-up. Any abnormalities should prompt a referral to a cardiologist for a thorough assessment. Patients should be counseled on ways to maintain heart health, including eating a well-balanced diet, getting regular exercise, and avoiding tobacco smoke. Additionally, practitioners should monitor for controllable risk-factors of cardiac disease, including cholesterol and blood pressure, at regular intervals.
Genitourinary dysfunction
Although most children who have undergone HSCT do not develop significant renal dysfunction, a retrospective study of 121 long-term survivors found 24% with measurable decreases in the glomerular filtration rate (GFR). [112] Despite this high prevalence, only 4% had severe renal dysfunction with GFR of less than 70 mL/min/1.73 m2. Those children who develop kidney disease following HSCT develop one of two forms: insidious, worsening renal failure as a consequence of exposure to nephrotoxic medications (such as alkylators, aminoglycosides and antifungals), radiation, infections, or GVHD; or acute renal dysfunction associated with hemolytic anemia. [113, 114]
Although renal dysfunction likely plateaus one year from transplant, assessing the serum creatinine levels in children who are long-term survivors of HSCT at entry into a long-term follow-up program is important. If any elevation of creatinine levels are noted, an assessment of GFR and referral to a nephrologist is warranted.
GI dysfunction
The primary gastrointestinal long-term complications of HSCT include intestinal dysfunction as a consequence of GVHD, liver dysfunction as a sequelae to acute liver damage (eg, due to sinusoidal obstructive syndrome [SOS] or acute GVHD), or late-onset liver damage secondary to viral infections or iron overload. Although the risk of acquiring blood-borne hepatitis B or C has been markedly reduced in the era of blood screening, [115] long-term survivors of HSCT may have been exposed prior to the development of screening processes.
Iron overload has not been well-studied in survivors of childhood HSCT, although iron overload pre-transplant has been associated with a higher treatment related mortality and a higher rate of SOS. [116] Because of the known complications of iron overload in patients receiving chronic transfusions, damage to the liver in patients with high transfusion requirements during HSCT is possible. A recent study demonstrated that children with high-risk acute lymphoblastic leukemia often receive large transfusion volumes during treatment, [117] suggesting that relapsed patients coming to HSCT may have undiscovered, premorbid iron overload heading into transplantation.
In addition to a thorough history and physical examination to elicit any GI signs or symptoms, all patients who have undergone HSCT should have liver function tests performed upon entry into long-term follow-up. Any abnormalities should prompt a referral to a gastroenterologist for a thorough assessment. Patients should be counseled on ways to maintain liver health, including eating a well-balanced diet and avoiding excessive alcohol consumption. Iron overload can be assessed by serum ferritin measurement. For those at high risk for iron overload, it can be assessed by noninvasive techniques such as iron-quantifying MRI technology.
Hematologic dysfunction
Chronic GVHD can manifest as autoimmune and alloimmune hemolytic anemias (AIHA) following HSCT, most commonly following unrelated-donor HSCT and in those undergoing HSCT for nonmalignant diseases. [118]
Dental complications
Conditioning regimens for HSCT can cause severe damage to developing teeth. Hypoplasia, microdontia, thinning of roots, and increased caries have all been demonstrated in survivors of HSCT during childhood. [119, 120] In a recent study of 27 pediatric patients, a statistically significant increase in root stunting was observed in those who had undergone HSCT, increasing the risk of lost teeth. [121]
At entry into long-term follow-up, counsel patients on good oral hygiene, including brushing with a soft tooth brush, flossing, and twice annual dental visits and cleaning.
Ocular complications
Visual defects are common in children after HSCT. In a study of 100 children surviving more than 5 years after HSCT, almost one third had long-term visual defects. [122]
Cataracts are the most common ocular complication of HSCT in children and are usually associated with radiation. Indeed, cranial radiation (including TBI) and GVHD represent the greatest risk factors for the development of cataracts. [123] The incidence of cataract development after TBI is less after hyperfractionated TBI than after single-dose TBI (13% vs 21% p < 0.001). [124] The cataracts after TBI are posterior and subcapsular compared with nuclear cataracts that occur with old age.
Ocular manifestations of chronic GVHD include keratoconjunctivitis sicca, as discussed above. For patients with exposure to ocular radiation, including TBI, and corticosteroids, long-term follow-up should include annual evaluations by an eye doctor with a comprehensive examination, including dilation and slit lamp evaluations.
Audiologic complications
Patients who received radiation to the ear (including TBI); those conditioned with ototoxic medications such as cisplatin, carboplatin, or thiotepa; and those who received ototoxic supportive care such as extensive aminoglycosides are at increased risk for hearing loss. This tends to be sensorineural hearing loss, affecting the high frequencies first. A study of 235 children and adolescents who received HSCT reported a 2-3% incidence of hearing loss after a median 11 years of follow-up. [125]
All patients exposed to TBI or ototoxic medications should have a hearing test performed at entry into long-term follow-up.
Skeletal complications
Osteonecrosis, reduced bone mineral density, and avascular necrosis (AVN) can occur in long-term survivors. These complications are usually secondary to high-dose steroids, radiation therapy, or estrogen depletion. The biggest risk factors for the development of AVN include corticosteroids and radiation, especially TBI. [126] The highest risks of AVN from TBI occur with a single dose of 10 Gy or higher or fractionated doses of 12 Gy or higher. [127] The average time to onset of AVN is 18 months from HSCT. Patients who develop avascular necrosis after HSCT can receive joint replacements, [128] although a limited joint lifespan for prosthesis is noted, typically 15-20 years. Given that survivors of childhood HSCT are likely to require their joints for far longer than that, early joint replacements can necessitate additional surgeries later in life.
All patients entering long-term follow-up who have received TBI or corticosteroids should have a careful history and physical examination focusing on the joints, especially the hips. Pain is the most common presenting sign of AVN.
Psychosocial and Cognitive Complications of Bone Marrow Transplantation
Children receiving hematopoietic stem cell transplantation (HSCT) are at high risk for psychological problems as a consequence of many factors, including chemotherapy, radiation therapy, prolonged isolation away from home, feelings of guilt about consuming the family's resources and body image. Posttraumatic stress disorder (PTSD), anxiety, and depression have all been reported in survivors of HSCT during childhood, with a prevalence rate as high as 35%. [129, 130]
In terms of family stress, siblings of patients undergoing HSCT, both donor and nondonor may also have posttraumatic stress reactions, feelings of guilt if the HSCT does not cure the recipient, and lower self-esteem than that of control subjects. Siblings of patients with HSCT who are not selected to donate also have evidence of low self-esteem. [131, 132] Several investigators have also observed psychosocial distress in parents of children undergoing HSCT. This distress can include anxiety as well as PTSD, especially in mothers. [133] Family factors are also important predictors of child adjustment during HSCT. [134]
Some studies suggest that children with cancer treated with CNS-directed chemotherapy or cranial radiation can develop neurocognitive dysfunction, including changes in attention and processing speed. [135, 136] Despite the concerns these findings may raise for survivors of childhood HSCT, a study of 54 survivors of HSCT during childhood showed no change in global measures of intelligence or academic achievement at 3 years from HSCT. However, subgroup analysis showed that patients transplanted prior to age 6 years, and especially those transplanted prior to age 3 years, are at risk for cognitive decline. [137]
For children undergoing HSCT, perform neurocognitive testing prior to HSCT and then again at entry into long-term follow-up and as needed as the child progresses through school. The findings obtained from a thorough neurocognitive assessment can be used to generate an individualized education plan through the school district, enabling the child for success by bolstering any weaknesses while allowing the child’s educational strengths to compensate for any deficits. This sort of intervention can impact the child’s school performance, thus impacting employment and overall quality of life.
Implications for Follow-up Care
The most important long-term complications in survivors of hematopoietic stem cell transplantation (HCST) include infection secondary to immune suppression, secondary malignancies, endocrinopathies, cardiac or pulmonary dysfunction, and cognitive and psychological sequelae. Thus, physicians should be well versed in monitoring for these complications and in providing prevention and treatment for these complications when possible.
The table below shows guidelines for screening for these problems.
Table 1. Early Identification of Complications in Long-Term Survivors of Hematopoietic Stem Cell Transplantation [9] (Open Table in a new window)
Complication |
Measures for the Physician |
Measures for the Patient |
Immunodeficiency |
|
|
Chronic GVHD |
|
|
Secondary Malignancies |
|
|
Endocrinopathies |
|
|
Pulmonary |
|
|
Cardiac |
|
|
Renal |
|
|
Dental |
|
|
Ocular |
|
|
Auditory |
|
|
Reduced bone mineral density |
|
|
Avascular necrosis |
|
|
Learning impairment |
|
|
GI complications |
|
|
Neurologic |
|
|
Psychosocial adjustments |
|
|
Changes in general health |
|
|
Summary
The long-term care of survivors of hematopoietic stem cell transplantation (HSCT) is multisystem and complex, requiring coordinated care across disciplines. The complications can be related to the underlying disease for which transplantation is performed, prior therapy, conditioning regimens, graft versus host disease (GVHD), or immunosuppression. Awareness of these potential risks is the first step toward preventing and treating them.
After the risks are identified, important preventive measures or treatment modalities may begin. Specific preventive-care measures include vaccinations, awareness of preventive/supportive programs, screening for secondary malignancies, and assessing for cognitive, psychosocial and organ function.
-
This bone marrow film at 400X magnification demonstrates a complete absence of hemopoietic cells. Most of the identifiable cells are lymphocytes or plasma cells. Photographed by U. Woermann, MD, Division of Instructional Media, Institute for Medical Education, University of Bern, Switzerland (http://www.aum.iawf.unibe.ch/).
Tables
What would you like to print?
- Overview
- Immunosuppression and Graft Versus Host Disease After Hematopoietic Stem Cell Transplantation
- Secondary Malignancies After Hematopoietic Stem Cell Transplantation
- Physical Complications After Bone Marrow Transplantation
- Psychosocial and Cognitive Complications of Bone Marrow Transplantation
- Implications for Follow-up Care
- Summary
- Show All
- Tables
- References