Background
Cervical spine (C-spine) injuries are relatively uncommon in sports but can be devastating when they occur. [1] Over the past few decades, the incidence of catastrophic C-spine injuries in sports has decreased significantly. This decrease is the result of monumental rule changes, such as the ban on spearing in American football, better coaching on contact and tackling techniques, the presence and instruction of athletic trainers at all levels of play, and the improvement in protective gear (eg, helmets and shoulder pads). Unfortunately, when catastrophic neurologic injuries do occur, they are permanent and life-changing.
Well over half of catastrophic injuries in sports are C-spine injuries. These injuries have been reported in most contact sports, including football, hockey, rugby, wrestling, and ice hockey, [2] as well as in several noncontact sports, such as skiing, track and field, diving, surfing, power lifting, paragliding, [3] and equestrian events.
Of all sports, football and rugby have the highest incidence of C-spine injuries. [4, 5, 6] Injury usually is secondary to high-velocity collisions between players, causing acceleration or deceleration of the head on the neck. [7] Acceleration usually causes a whiplash type of extension force on the neck, whereas deceleration usually results in flexion forces. [8]
C-spine injuries are estimated to occur in 10-15% of all football players, most commonly in linemen and defensive players. Serious injuries with neurologic sequelae remain infrequent, and most of these injuries are self-limited. Injuries occur in all levels of play, from the high school to the professional level. [9, 10, 11, 12, 13, 14]
Spearing, which has been banned in American football since 1976, occurs when a player uses the helmet/head as the first point of contact with another player. Spearing is a significant cause of C-spine injuries and quadriplegia. The force transmitted to the cervical spine in these cases is one of axial compression with the vertebrae in positions of slight flexion. [15]
The natural architecture of the normal C-spine assumes a lordosis of the vertebrae. This lordosis allows for controlled motion and the transmission of forces to the supporting muscles and soft tissues. When the neck is slightly (~30°) flexed, the normal lordosis is straightened, and the forces of the axial load are transmitted to the bones and disks. If the impact force is greater than the yield strength of the vertebrae, a fracture and possible dislocation with spinal cord injury (SCI) can occur. [16, 17]
One of the most challenging roles of the team physician involves addressing C-spine injuries in contact sports. It is essential that he or she be well versed in the prevention, evaluation, stabilization, and treatment of these injuries. [18] A high index of suspicion and an understanding of cervical alignment and architecture, as well as comprehension of the mechanics exerted during a sporting event, are imperative for diagnosing cervical injuries. [19, 20]
The team physician plays a crucial role in the coordination of medical assessment on the playing field, immobilization and transportation to a qualified facility for evaluation and treatment, and decision-making regarding return to play following an injury. These decisions should be discussed with the athlete and the athlete's parents, coaches, trainers, and agents. The ultimate decision should be made in the best interest of the patient. [21]
Although professional athletes are more likely to sustain more severe injuries and require surgical treatment than high school or college athletes are, [22] C-spine injuries are an issue in the pediatric population as well. A study of C-spine injury and SCI in children (aged 4-9 years), preadolescents (aged 10-13 years), and adolescents (aged 14-17 years) found that the incidence of these injuries increased with age, raising concerns about youth sports played at a competitive level. [23]
For patient education resources, see the First Aid and Injuries Center as well as Whiplash.
Spectrum of injury
Cervical injuries that result from participation in sports usually are self-limited and can be divided into the following categories:
-
Nerve root or brachial plexus injuries
-
Acute cervical sprains/strains
-
Intervertebral disk injuries
-
Cervical fractures and dislocations [24]
-
Cervical stenosis
Imaging considerations
The optimal imaging strategy for the spine is often unclear. Conventional imaging was long the primary imaging modality of choice for the cervical spine, but evidence has been published to suggest that multidetector computed tomography (CT) may be preferable for cervical spine injury. [25] However, this may not be the case for children and adolescents.
Hannon et al conducted a study to determine the optimal means of evaluating pediatric patients with cervical spine injury from blunt trauma. In patients requiring imaging, screening radiography was the preferred modality in conjunction with focused CT. [26] CT was preferred when the probability of cervical spine injury was 24.9% or higher. The investigators concluded that in a hypothetical pediatric trauma population, clearing and screening radiographs are preferred for evaluation and that CT is rarely optimal for initial evaluation.
Nerve Root or Brachial Plexus Injuries
The most common cervical injury in football players involves transient loss of function with searing or lancing pain down one arm following a collision. These injuries are colloquially referred to as stingers or burners. [27, 28, 29] Prospective studies performed at Tulane University showed a 7.7% incidence of stingers in a group of college football players.
Initially, the player complains of total arm weakness and a radiating burning sensation that usually resolves. Numbness in the C6 dermatomal distribution may persist. Motor weakness of shoulder abductors, elbow flexors, external humeral rotators, and wrist and finger extensors also may persist. The duration of symptoms ranges from 2-10 minutes to 24 hours. Symptoms are reproduced by the Spurling maneuver. Function gradually returns from the proximal muscle groups to the distal muscle groups.
The severity of the injury correlates with the underlying pathophysiology. Neurapraxia is a selective demyelination of the nerve sheath, and it is the most benign injury. Axonotmesis is a disruption of the axon and the myelin sheath, with the epineurium remaining intact. The most severe injury is neurotmesis, which is a compete disruption of the endoneurium; this injury is associated with the most unfavorable prognosis.
The differential diagnoses of burners include acute cervical disk herniations, foraminal stenosis, and extradural intraspinal masses.
Stingers are thought to be the result of either of the following mechanisms:
-
A distraction or stretch injury in which the head is driven to the side opposite the painful arm and the ipsilateral shoulder is depressed; this causes a momentary stretch injury to the upper cords of the brachial plexus
-
Compression and rotation of the extended cervical spine toward the painful arm, with injury occurring because the cervical nerves are tethered by fibrous tissue between the vertebral arteries and the distal foramina at each cervical level; these dentate ligament attachments become taut and stretch the cervical nerve roots as they leave the spine
Because most burners are self-limited, the most important treatment obligation of the team physician is to rule out an unstable cervical injury. The key to assessment is that patients with burners have full pain-free neck range of motion (ROM). If neck motion is decreased or painful, withdraw the athlete from play and obtain cervical radiographs to rule out fracture/dislocation. If symptoms persist for 3-4 weeks following injury, perform electromyography (EMG) to evaluate upper-trunk function. [30]
Acute Cervical Sprains or Strains
A sprain is defined as an injury to the paraspinal musculotendinous unit. A strain is defined as an injury to the paraspinal muscle itself. An athlete with a sprain or a strain usually presents after jamming his/her neck. The pain is localized to the C-spine and limits cervical ROM. Pain and paresthesias do not radiate to the arms. The neural examination is normal, and radiographs are negative for evidence of fracture or dislocation.
Take the player through a series of movements to evaluate the ROM. If the patient has full ROM with no radiation of pain, no paresthesias, and a normal neurologic examination, treat with nonsteroidal anti-inflammatory drugs (NSAIDs) and a soft cervical collar (C-collar) for comfort. Once the pain has resolved, the athlete can return to activity. If the player has limited ROM, protect the neck and remove him or her from activity.
Obtain a radiographic series to include anteroposterior (AP) and lateral flexion/extension views. If a fracture or dislocation is encountered, institute proper immobilization and stabilization. If these radiographs are negative but symptoms persist, obtain magnetic resonance imaging (MRI) to rule out disk herniation.
Intervertebral Disk Injuries
Acute disk herniations in football are rare. However, with the acute onset of transient neurologic deficits and negative cervical radiographs, the possibility of a ruptured cervical disk must be considered. Symptoms of herniation vary from radiculopathy to anterior cord syndrome. Anterior cord syndrome occurs with an acute paralysis of the upper, the lower, or all four extremities. An associated loss of pain and temperature sensation to the level of the lesion occurs. [31] The posterior-column vibratory, proprioceptive, and light touch sensations are preserved.
A high degree of clinical suspicion is necessary to avoid missing the diagnosis of a disk injury. If disk injury is suggested, confirm the diagnosis with CT myelography (see the first and second images below) or MRI (see the third and fourth images below). Once the diagnosis of acute disk herniation with neurologic symptoms is made, an anterior diskectomy with interbody fusion may be necessary.
In contrast to the rarity of acute disk herniations in contact sports, disk injuries without frank herniation or neurologic injury can be common and are characterized by chronic changes. Chronic disk changes frequently are seen in athletes who compete in contact sports. Albright studied cervical spine radiographs of 75 University of Iowa freshmen football recruits. [32] The radiographs were obtained after the individuals played high school football but before they played college football. Albright found that 32% had one or more of the following conditions:
-
Occult fracture
-
Vertebral compression fracture
-
Disk-space narrowing
-
Osteophytes and degenerative changes
Albright's findings illustrate that the constant loading of the C-spine in contact sports leads to chronic degeneration. MRI of patients with chronic disk injuries reveals a disk bulge with no obvious herniation. Treatment of these patients is conservative, and contact activity should be withheld until the athlete has regained painless full ROM of the C-spine.
Cervical Fractures and Dislocations
Cervical fractures and dislocations occur when the axial loading forces applied to the C-spine are greater than the yield strength of the vertebral bodies or the supporting ligamentous structures. A spectrum of pathology exists, including the following:
-
Subluxation without fracture, with or without neurologic injury
-
Dislocations, with or without neurologic injury
-
Fractures, with or without neurologic injury
The most important consideration in discussing cervical fractures and dislocations is the concept of stability. Stability, as described by White et al, is the ability of the spine to limit its patterns of displacement during physiologic loads so as to prevent damage to or irritation of the spinal cord and nerve roots. [33] Instability of the adult spine therefore is defined as dysfunction of the posterior elements with more than 3.5 mm displacement (or >20% translation) in the horizontal sagittal plane. (See the image below.)
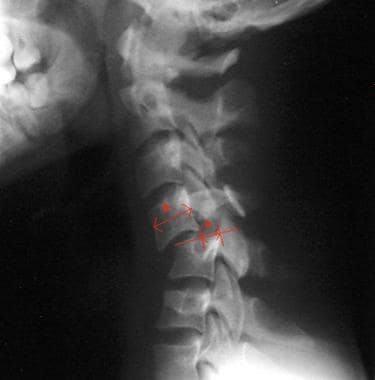
Instability is also apparent by analyzing the angular measurements between motion segments. Sagittal plane rotation exceeding 20° on flexion/extension films is considered abnormal and potentially unstable. In the acute setting where flexion/extension radiographs are not obtainable, more than 11° of relative sagittal plane angulation between cervical motion segments on a static lateral C-spine radiograph is considered unstable. (See the image below.)
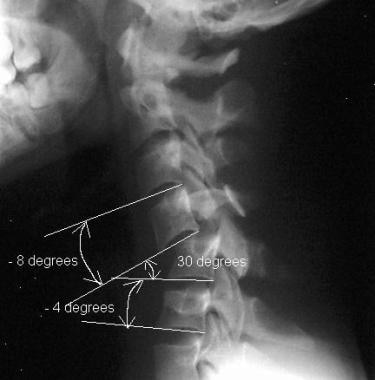
White and Panjabi's work on cervical instability culminated in the creation of a scoring checklist to serve as an algorithm and an objective assessment of instability (see the image below). Patients are assessed anatomically, radiographically, neurologically, and physiologically. The patient is graded on each of these criteria, and the grades are added to obtain the final score. A total score of 5 or more is indicative of a patient with an unstable spine.
Subluxation or dislocation without fracture results from disruption of the posterior soft-tissue supporting elements. Angulation and anterior translation of the superior vertebrae occurs. No associated fractures exist, and associated neurologic injuries may or may not be present. The diagnosis is made by flexion/extension lateral C-spine radiographs, which show active motion, anterior intervertebral disk space narrowing, and fanning of the spinous processes posteriorly. It is imperative that these radiographs be obtained in the presence of the treating spine surgeon and in a patient who is awake and communicative (see the images below).
An alternative to the flexion/extension radiographs is the use of controlled axial-traction lateral radiographs, also referred to by White as the "stretch test." [34] The stretch test is performed after a standard nontraction lateral C-spine radiograph has been obtained and examined to rule out obvious instability or subluxation.
In the stretch test, the patient is placed supine with the head supported on a roller platform to reduce friction. The head is placed in a traction rig with either Garner-Wells tongs or a head halter, and 10-lb (4.5-kg) weight loads are applied incrementally. The 10-lb weights are added in the presence of the treating physician. The maximum weight allowed is equivalent to 33% of the patient's body weight.
The physician performs serial neurologic assessments of the patient with each addition of weight. A lateral C-spine radiograph is obtained with each addition of weight. The time interval between weight increments should be at least 5 minutes. The stretch test is considered positive for instability if one of the following occurs:
-
The patient sustains a change in neurologic function
-
On comparison with the pretraction radiograph, there is more than 1.7 mm of interspace separation of the anterior or posterior elements
-
There is a change of greater than 7.5° in the angle between vertebrae
The prognosis in patients with subluxation without fracture depends on the degree of displacement. Instability is likely despite nonoperative treatment, and if anterior subluxation is more than 20% of the vertebral body width, treatment should be posterior cervical fusion.
Atlanto-occipital dislocations (see the image below) result from high-speed collisions and have never been reported in football. They are described as complete injuries. These injuries usually are fatal secondary to complete respiratory arrest. When this injury is suspected, cervical traction is contraindicated. If the patient survives the injury, treatment is to align the spine and place the patient in a halo vest until C0-C2 fusion can be performed.
A traumatic rupture of the transverse ligament of the atlas (see the images below) leads to widening of the atlantodens interval (ADI) and decreased space for the spinal cord.
C1 (atlas) fractures
C1 or atlas fractures (see the images below) usually result from axial loading and are decompressive fractures that rarely result in neurologic deficits. They can be classified as follows:
-
Anterior-arch fractures
-
Posterior-arch fractures
-
Lateralmass fractures
-
Jefferson (burst) fractures
All C1 or atlas fractures can be treated with halo vest immobilization until fracture healing occurs.
Odontoid fractures
Odontoid fractures are classified into three types as follows.
Type I
Type I odontoid fractures are cephalad to the transverse ligament and are secondary to avulsion of alar ligaments. They rarely are associated with neurologic injury or C1-C2 instability. Stability can be assessed with lateral flexion/extension views. Most type I odontoid fractures can be treated with a cervical collar (C-collar).
Type II
Type II odontoid fractures (see the image below) are through the neck of the odontoid process. These fractures usually are secondary to hyperextension, flexion, or rotational forces.
The overall union rate of type II fractures is 68% with halo treatment. Type II odontoid fractures with more than 10° of angulation or more than 5 mm of translation should be treated with surgery rather than a halo to decrease the pseudoarthrosis rate.
Nonunions can be common among type II odontoid fractures. In patients with significant risk factors, primary C1-C2 fusion by a variety of techniques should be employed. Patients with low risk factors for nonunion can be treated with reduction and halo immobilization for 12 weeks. The risk factors for nonunions include the following:
-
Age older than 50 years
-
Displacement of more than 5 mm
-
Posterior versus anterior displacement
-
Excessive halo vest treatment
Type III
Type III odontoid fractures (see the images below) extend into the cancellous bone of the body of C2 and therefore have a high rate of union. These fractures usually can be treated with halo immobilization for 12 weeks. If the fracture is impacted and has a stable pattern, treatment with a rigid C-collar in a compliant patient for 12 weeks can be an option.
Traumatic spondylolistheses of C2 (axis)
Traumatic spondylolistheses of C2 (axis) also can occur. These fractures also are known as hangman's fractures. They usually are diagnosed on lateral C-spine radiographs. Neurologic injury usually does not occur unless a C2-C3 facet dislocation is present. Traumatic spondylolistheses of C2 may be divided into four types as follows.
Type I
A type I hangman's fracture (see the image below) is either nondisplaced or has less than 3 mm of C2-C3 translation. No angulation of the fracture fragments exists. The injury occurs secondary to a combined hyperextension/axillary compression force. This type of fracture usually can be treated with a C-collar for 3 months.
Type II
Type II fractures are associated with significant translation of more than 3 mm with angulation. These injuries are secondary to initial hyperextension-loading forces, followed by combined flexion/compression forces. Treatment for type II injuries depends on the amount of initial translation, as follows:
-
For 3-6 mm of initial translation, treat with a halo vest for 3 months
-
For more than 6 mm of translation, treat with 4-6 weeks of halo traction, followed by halo vest immobilization for 3 months
Type IIA
This injury also is known as the Starr-Eismont variant (see the image below) and is associated with significant angulation and minimal translation. Traction in these injuries is contraindicated because it leads to significant translation and associated neurologic injury. Treatment includes immediate halo vest with mild axial compression for reduction.
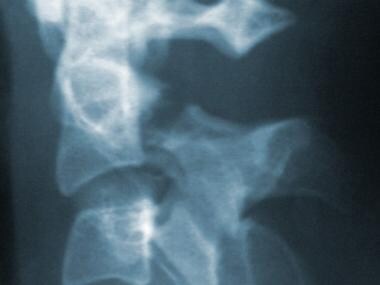
Type III
Type III injuries are associated with severe angulation and translation. Unilateral or bilateral facet dislocations usually accompany them. The mechanism of injury is a combined flexion/compression force. Closed reduction is exceedingly difficult. Open reduction with internal stabilization usually is required.
Injuries of the subaxial cervical spine generally are associated with an increased likelihood of neural compression because of a decreasing ratio of canal diameter to cord diameter.
Types of injuries
Avulsion fractures
The spinous process is the usual location of avulsion fractures. When these fractures occur at C7 (see the image below), they are termed clay shoveler's fractures. This injury results from forceful contraction of trapezius and rhomboid muscles or from a sudden severe flexion force transmitted to posterior spinous ligaments. Treat with a C-collar for comfort.
Compression fractures
Compression fractures are defined as the vertebral body having an intact middle column and loss of anterior body height. Evaluate compression fractures with flexion and extension radiographs, CT, and MRI. (See the images below.)
Treatment depends on the degree of anterior compression, as follows:
-
If there is less than 25% anterior compression, treat the patient with a C-collar
-
If there is more than 50% anterior compression, patients often have posterior ligamentous failure, which results in significant instability that requires posterior fusion with or without anterior column reconstruction
Facet joint injuries
These subluxations and dislocations occur as a result of disruption of the supraspinous ligaments, interspinous ligaments, ligamentum flavum, and facet capsule. Facet joint injuries can be unilateral or bilateral, and neurologic injury varies. Lateral radiographs help the physician confirm the diagnosis. CT helps rule out bony involvement, and MRI delineates associated disk herniations. (See the images below.)
The mechanism of injury corresponds to whether the lesion is unilateral or bilateral. Unilateral lesions are the result of flexion and rotation with axial force. Bilateral lesions are the result of severe flexion with axial loading.
Treatment is controversial because of the risk of further neurologic injury from further disk herniation with the reduction maneuver. The goals of treatment are as follows:
-
Prevent further neurologic injury
-
Reduce the subluxation/dislocation
-
Stabilize the spine in the reduced position
The focus of controversy is the question of whether to obtain an MRI before performing a closed reduction of the subluxation or dislocation. Eismont recommended MRI prior to reducing a subluxation to rule out a herniated nucleus pulposus that could further herniate during the reduction and result in catastrophic cord sequelae. [35] Cotler et al suggested that high-weight rapid reduction should be performed as soon as possible and that MRI should be performed following the reduction.
Vaccaro et al, in a prospective study of 11 patients with cervical dislocations who underwent prereduction and postreduction MRI, found that disk herniations were identified in two of the 11 before reduction. [36] They performed awake closed reduction by high-weight rapid traction. Reduction was successful in nine of the 11 patients. Of the nine patients with successful closed reduction, two had disk herniations before reduction, and five more had disk herniations on the postreduction MRI. None of the patients had neurologic worsening after reduction.
Cotler and Vaccaro therefore concluded that MRI can be done after the subluxation or dislocation is reduced to assess the cord and disk. In cases of acute paraplegia, the moments saved can be cord-saving.
Eismont agreed with this last point in patients with complete distal motor loss, with or without distal sensation (Frankel grades A and B), on the grounds that these patients have the most to gain and least to lose by rapid reduction and the possible associated risks. [35] Eismont did not agree in the circumstance of patients who are neurologically intact or near normal (Frankel grade D and E), on the grounds that these patients have the most to lose in the event of further disk herniation with closed reduction leading to paralysis.
Eismont recommended provisional immobilization with a rigid C-collar, 10 lb (4.5 kg) of skull traction, and MRI before reduction is attempted. [35] If no disk herniation is detected, reduction is performed with skeletal traction of 5-7 lb (2.25-3.2 kg)/level (maximum, 50 lb [22.5 kg]); radiographic results and neurologic function are closely monitored during reduction. Once the reduction is obtained, posterior fusion should be performed if there is no associated disk herniation. If a disk is encountered on the prereduction MRI, the recommended treatment consists of anterior cervical diskectomy, reduction, and plate fixation.
Vertebral burst fractures
Cervical burst fractures result from severe axial loading in combination with hyperflexion forces. Burst fractures include a comminuted fracture of the middle spinal column, and if they are destabilized posteriorly, they can result in kyphosis. Cord injury usually is present because of retropulsion of fracture fragments.
Perform a radiographic series, CT, and MRI to evaluate severity of neural compression, intramedullary cord injury, and ligamentous damage. Employ skeletal traction for spinal realignment and decompression of the canal by ligamentotaxis of retropulsed fragments.
Perform an MRI with the patient in traction to assess the severity of canal compromise post reduction. In patients with canal compromise and neural defects, perform an anterior decompression and reconstruction with strut graft and plate fixation. If the fracture is unstable (eg, a three-column lesion), the patient may require an anterior and posterior fusion/stabilization.
Teardrop fractures
Teardrop fractures result from severe flexion-axial loading forces and are three-column injuries. They are characterized by the following:
-
Displaced fracture of the anteroinferior corner of the superior body
-
Segmental disk disruption
-
Posterior ligamentous injury
-
Retropulsion of the proximal body into the neural canal
Radiographically, retrolisthesis of the posteroinferior portion of the involved body often results in neural compression with deficits ranging from nerve root injuries to complete spinal cord injuries. (See the images below.)
Diagnosis and treatment are the same as for burst fractures.
Risk for further injury
The issue of returning to play after a patient sustains a C-spine injury should be based on the risk for further injury.
Conditions with a slightly increased risk of reinjury following the initial insult include the following:
-
Asymptomatic bone spurs
-
Healed nondisplaced fractures
-
Stingers/burners
-
Healed disk herniations
-
Healed laminar fractures
-
Asymptomatic foraminal stenosis
Moderate-risk conditions that are associated with a significant chance for recurrence of symptoms and an increased risk for permanent injury include the following:
-
Facet fractures
-
Lateral-mass fractures
-
Nondisplaced healed odontoid fractures
-
Nondisplaced healed C1 ring fractures
-
Acute lateral disk herniations
-
Cervical radiculopathy secondary to foraminal spur
Extreme-risk conditions that have the highest risk of recurrence and of permanent damage include the following:
-
Ruptured transverse ligament of C1-2
-
Occipitocervical dislocation
-
Displaced odontoid fractures
-
Unstable fracture dislocations
-
Cervical cord anomalies
-
Acute central disk herniations
Cervical Spinal Stenosis
Cervical spinal stenosis is defined as the diminution of the AP diameter of the spinal canal, either as an isolated congenital observation or with disk herniation, degenerative changes, or posttraumatic instability. [37]
Methods of measuring the degree of spinal stenosis continue to evolve with the evolution of imaging technologies. In 1956, Wolfe et al described measuring the space for the cord on plain lateral C-spine radiographs by measuring the distance from the middle of the posterior surface of the vertebral body to the most anterior point on the spinolaminar line for C3-7. The normal range was 14.2-23 mm; less than 13 mm was considered stenosis. Values obtained by measurement on lateral plain radiographs could be skewed because of radiographic magnification or variations in radiographic techniques. The actual size of the canal is better assessed by means of CT.
To compensate for these variances in radiographic techniques, Torg and Pavlov described the Torg/Pavlov ratio. [38] This ratio is a measurement of the width of a given vertebral body on the lateral C-spine radiograph divided by the corresponding space allowed for the cord at the same level (see the image below). A value of less than 0.8 was considered to be cervical stenosis and a serious risk factor for neurologic injury in contact sports.
Herzog sugegsted that the Torg/Pavlov ratio may have resulted in false-positive indications. [39] In reviewing the CT scans of football players with abnormal Torg/Pavlov ratios, Herzog found that 70% of players with abnormally small ratios had normal-sized cervical spinal canals. These findings are explained by the abnormally large vertebral bodies found in football players. As a result, the denominator in the Torg/Pavlov ratio is larger and the ratio artificially decreased, resulting in a false positive. Additionally, Herzog found no correlation between a Torg/Pavlov ratio of 0.8 and any transient neurapraxia or permanent neurologic deficits.
Castro et al, in the American Journal of Sports Medicine, illustrated that cord diameter also varies and that it is the relative difference between the canal size and the cord diameter that creates the clinical condition of stenosis. [40]
Epstein concluded that the presence of a stenotic canal influenced the morbidity and prognosis of a spinal cord injury and that patients with the smallest anterior-posterior canal diameter had the most severe myelopathy following injury. [41]
Eismont et al echoed Epstein's conclusions when they looked at 98 patients with C-spine fractures and/or dislocations and found that the sagittal size of the cervical canal correlated with the extent of neurologic injury. [42] They concurred that patients with small diameter canals had more significant neurologic sequelae. Matsura et al found a correlation between the shape of the spinal canal, the central canal diameter, and the predisposition to spinal cord injury.
Cantu advocated the functional definition of spinal stenosis, [43] according to which functional spinal stenosis is present when the size of the canal is so small that the protective spinal fluid cushion around the cord is obliterated or when the cord is deformed on CT myelography or MRI. Any athlete with functional cervical stenosis is at increased risk for quadriplegia and should be prohibited from participating in contact sports.
Return To Play
To help establish objective guidelines for return to play following an injury, Watkins et al proposed a point grading system to quantify the patient's clinical situation. [44] This grading system was meant as a guideline for return to play. The physician must consider the entire scenario for each individual player. Watkins et al included the following three topics in their grading system:
-
Extent of neurologic injury
-
Time from injury to treatment
-
Narrowing of central canal diameter
For each of these, point values are assigned according to the player's condition, as follows.
The extent of the neurologic injury is graded as follows:
-
1 point - Unilateral arm numbness or dysesthesias or loss of strength
-
2 points - Bilateral arm loss of motor or sensory function
-
3 points - Ipsilateral arm and leg symptoms
-
4 points - Transitory quadriparesis
-
5 points - Transitory quadriplegia
The time from injury to treatment is graded as follows:
-
1 point - Less than 5 minutes
-
2 points - Less than 1 hour
-
3 points - Less than 24 hours
-
4 points - Less than 1 week
-
5 points - More than 1 week
The narrowing of the central canal diameter is graded as follows:
-
1 point - More than 12 mm
-
2 points - Between 12 and 10 mm
-
3 points - 10 mm
-
4 points - Between 10 and 8 mm
-
5 points - Less than 8 mm
The points tabulated from each of the three categories then are added together and assessed in terms of to the following scoring scale:
-
0-6 points - Minimal risk associated with return to play
-
6-10 points - Moderate risk associated with return to play
-
10-15 points - Severe risk associated with return to play
These criteria serve as a guide for the team physician; however, the physician should consider each case individually and request the appropriate consultations as needed. [45]
Professional athletes, being more likely to sustain more severe injuries and require surgical treatment than high school or college athletes are, typically have a longer wait before they can return to play. [22]
-
T1-weighted MRI of cervical disk herniation.
-
T2-weighted MRI of cervical disk herniation.
-
Axial CT scan of cervical herniated nucleus pulposus.
-
Myelogram of cervical herniated disk. Filling defect is shown.
-
Plain radiograph of jumped facets of C4 on C5.
-
Sagittal CT scan of jumped facet.
-
Sagittal MRI of facet dislocation of C7 on T1.
-
Jumped facets.
-
Jumped facet showing anterior displacement of one vertebra on adjacent inferior vertebrae.
-
Jumped facets with complete anterior displacement of proximal vertebrae.
-
Atlantooccipital dislocation.
-
Axial CT scan of increased atlantodens interval.
-
Sagittal CT scan reconstruction that shows widening of atlantodens interval.
-
Three-dimensional CT scan of C1.
-
Axial CT scan of Jefferson fracture.
-
Displaced type II odontoid fracture.
-
Anteroposterior view of type III odontoid fracture.
-
Coronal CT scan of type III odontoid fracture.
-
Type I C2 traumatic spondylolisthesis. Note anterior translation but lack of angulation.
-
Close-up lateral radiograph of type IIA (Starr-Eismont variant) C2 traumatic spondylolisthesis. Note significant angulation with minimal translation.
-
Avulsion fracture of C7 (clay shoveler's fracture).
-
C5 compression fracture.
-
Axial CT scan of C5 compression fracture. Intact middle and posterior columns are shown.
-
Teardrop fracture.
-
Posterior teardrop fracture.
-
Lateral cervical spine plain radiograph illustrating Torg/Pavlov ratio.
-
White and Panjabi Scoring Scale for Cervical Instability.
-
Distance (a) is measured from posterior-inferior corner of vertebral body above allegedly unstable disk space to posterior-superior corner of vertebral body below allegedly unstable disk space. Distance (b) is anterior-posterior sagittal-plane diameter of vertebral body above allegedly unstable disk space. According to White and Panjabi's outline, evidence of instability exists if distance (a) is greater than 20% of distance (b). Alternatively, if linear distance (a) is greater than 3.5 mm, instability is evident. In conclusion: If (a)/(b) x 100 > 20%, or if (a) > 3.5 mm, then instability is evident. In this example (a) = 5.5 mm, (b) = 13.0 mm. 5.5/13 x 100 = 42.3%, and 42.3% > 20%; therefore, instability is evident. Also, (a) = 5.5 mm, which is greater than 3.5 mm; therefore, instability is evident.
-
Schematic representation of White and Panjabi's description of abnormal angulation. Finding of abnormal angulation greater than 11° between supra-adjacent and subadjacent cervical motion segments on static lateral cervical spine (C-spine) radiograph is considered unstable. Basic mathematical formula to analyze this is as follows: Angle of motion segment in question minus angle of supra-adjacent segment or subadjacent motion segment. In normally stable C-spine, difference is less than 11°. In this image, formula is illustrated by following examples: For supra-adjacent level, 30 - (-8) = 38, and 38 > 11; for subadjacent level, 30 - (-4) = 34, and 34 > 11.