Overview
This article provides an overview of the various neurophysiological monitoring techniques used intraoperatively.
Intraoperative neurophysiological monitoring has been utilized in attempts to minimize neurological morbidity from operative manipulations. The goal of such monitoring is to identify changes in brain, spinal cord, [1, 2] and peripheral nerve function prior to irreversible damage. Intraoperative monitoring also has been effective in localizing anatomical structures, including peripheral nerves and sensorimotor cortex, which helps guide the surgeon during dissection.
Evoked potential monitoring includes somatosensory evoked potentials (SSEP), brainstem auditory evoked potentials (BAEP), motor evoked potentials (MEP), and visual evoked potentials (VEP). Electromyography (EMG) also is used extensively during operative cases. Scalp electroencephalography (EEG) provides data for analysis in SSEP, BAEP, and VEP. Scalp EEG also can be used to monitor cerebral function during carotid or other vascular surgery. In addition, EEG recorded directly from the pial surface, or electrocorticography (ECoG), is used to help determine resection margins for epilepsy surgery, and to monitor for seizures during electrical stimulation of the brain carried out while mapping cortical function.
Electrophysiological monitoring in the operative milieu poses several specific challenges. These include (1) presence of electromagnetic interference, and (2) use of anesthetic agents that can alter recordings.
History
In 1848, Du Bois-Reymond first demonstrated the action potential of nerves. He also is credited with describing the electrical activity of muscle, the first EMG. Electrical activity of the brain was described by Caton in 1875. Han Berger in 1928-29 was the first to report EEG tracings from human brains.
The first use of intraoperative EEG was by Foerster and Alternberger in 1935. In the late 1930s through the 1950s, Herbert Jasper and Wilder Penfield further developed this technique, using ECoG for localization and surgical treatment of epilepsy. [3] They also performed careful mapping of cortical function by direct electrical stimulation.
Dawson recorded the first SSEP in 1947. [4] Understanding of other evoked potentials, including those produced by motor activity and by visual and auditory stimulation, followed. In 1978, the first intraoperative use of BAEP was reported. Since then the following refinements in recording techniques have taken place:
-
Improved differential amplifiers with development of transistors and integrated circuitry
-
Reduced size of equipment to allow more widespread intraoperative use
-
Signal-averaging techniques to allow recording of small signals on a large electrical background
-
Computerized equipment with microprocessors to allow multiple channel recordings and various filtering capabilities as well as spectral analysis
-
The image below depicts normal brainstem auditory evoked potentials
Electromyography
Purpose of Electromyography
EMG is the recording of electrical activity of muscle. Changes in EMG recordings are indirect indicators of function of the innervating nerve. Intraoperative uses have stressed localization and assurance of the integrity of peripheral nerves, including cranial nerves.
Technique
Multiple EMG needles typically are placed into the muscles to be examined. Practically any muscle can be monitored, including face, tongue, and sphincter musculature. EMG is recorded continually with a low noise amplifier. Recordings are displayed visually and usually also sent to a speaker to provide auditory feedback. Changes in muscle electrical activity then can be seen and heard. When a peripheral nerve is to be localized intraoperatively, a sterile stimulating probe is used during the operation. The image below illustrates two types of EMG needles.
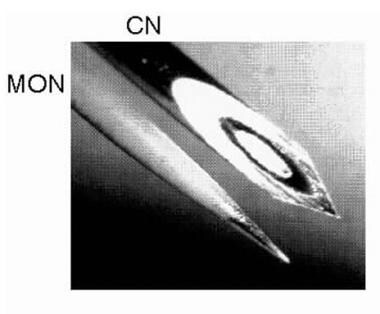
Interpretation
Spontaneous or induced EMG activity is monitored. Additionally, direct electrical stimulation of the nerve can help localize the neural structure. Note that spontaneous EMG activity does not assure the integrity of the peripheral nerve. If evoked EMG activity can be elicited consistently, integrity of the distal nerve and muscle can be assured.
Clinical Uses
Facial nerve/other cranial nerve monitoring
Cranial nerve monitoring is useful for surgical procedures in which the facial nerve is at risk, including posterior fossa surgery (eg, acoustic neuroma), vestibular neurectomy, surgery in the temporal bone, and parotid gland surgery. [5] Trigeminal, glossopharyngeal, vagus, spinal accessory, and hypoglossal nerve functions can be monitored similarly by EMG. Electrical stimulation in the operative field can evaluate the integrity of peripheral nerves. Spontaneous EMG activity suggests manipulation in the vicinity of the cranial nerve.
Selective dorsal rhizotomy
Selective dorsal rhizotomy (SDR) is a procedure that is used to reduce debilitating spasticity in conditions such as cerebral palsy by selectively transecting spinal rootlets. Overactive excitatory influence on motor nerves is believed to be reduced by removing facilitory afferent input from muscle spindles. The procedure consists of stimulating spinal rootlets and monitoring EMG and motor function. Those rootlets that are associated with an abnormal motor response are sectioned selectively.
Tethered spinal cord release
Patients who undergo a tethered cord release procedure require dissection of scar tissue and possibly section of the filum terminale. Distinguishing functional neural elements from nonfunctioning tissue is important. Stimulation of the roots of normally functioning nerves in the cauda equina elicit EMG activity. Monitoring lower extremity musculature, as well as anal and urethral sphincters, is important if the sacral roots are involved.
Pedicle screw placement
Use of pedicle screws as a fixation device in posterior spinal instrumentation in the lumbar region has become increasingly common. Various techniques to assure correct placement of the screws are advocated currently. EMG monitoring makes use of the fact that improperly placed screws that broach the cortical confines of the bony pedicle or vertebral body cause low impedance between the screw and the exiting nerve root underneath the pedicle. Properly placed screws that remain entirely within the bone have high impedance. If the screw is stimulated with constant voltage greater than 30 volts (V) without EMG activation, the screw is unlikely to have broached the vertebral cortex. However, a response to stimulation at less than 20 V suggests a bony defect that provides a low impedance pathway to the nerve root.
Evoked Potentials
Somatosensory Evoked Potentials
Technique
SSEPs are recorded by stimulating peripheral afferent nerves, usually electrically; they are recorded with the help of scalp electrodes. Because of the presence of nonspecific EEG background activity, the evoked potential must be averaged to improve signal-to-noise ratio.
In intraoperative use, the median nerve at the wrist is the most common stimulation site for upper extremity monitoring. In the lower extremity, the posterior tibial nerve just posterior to the medial malleolus is used most commonly. Other sites that can be utilized include the ulnar and peroneal nerves. [6]
Needle electrodes generally are used to reduce artifactual signals. Recording electrodes are placed on the scalp and on the cervical spine. [7] Additionally, electrodes can be placed at the Erb point for upper extremity SSEP recording and over the lumbosacral spine for lower extremity recording.
If the operative field affords exposure, recording electrodes may be placed directly in the epidural space. Typically, the electrodes are placed just proximal to the lesion of concern.
Operating rooms are inundated with equipment that emits electromagnetic interference, which is greatest at the frequency of alternating current (60 Hz in the United States). Adequate filtering to remove this artifact is important. Additionally, shielding to reduce interference is essential.
Interpretation
Amplitude, shape, and latencies of the responses are monitored. Serially recorded responses are compared with laboratory norms. Establishing a reproducible baseline recording prior to any positioning or surgical manipulations is important (see the image below). Changes from the baseline responses are the most important indicators of neurological dysfunction. Keep in mind that anesthetics can alter the evoked responses significantly.
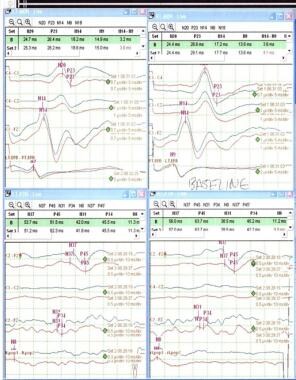
Typical responses to median nerve stimulation are shown in Table 1.
Table 1. Typical Responses to Median and Tibial Nerve Stimulation (Open Table in a new window)
Name |
Recording Site |
Latency |
Probable Anatomical Location |
Median nerve |
|
||
Erb point |
Erb point |
9 ms |
Trunks of brachial plexus |
Cervical A |
Cervical |
11 ms |
Dorsal root entry zone of cervical roots |
Cervical B |
Cervical |
12-13 ms |
Posterior columns |
Cervical C |
Cervical |
14 ms |
Brain stem |
N18 |
Scalp |
18 ms |
Subcortical structures |
N20 |
Scalp |
20 ms |
Somatosensory cortex |
Tibial nerve |
|
||
N20 |
Lumbar |
20 ms |
Spinal roots/cord |
P27 |
Cervical spine |
27 ms |
Nucleus gracilis |
N35 |
Scalp |
35 ms |
Somatosensory cortex |
P40 |
Scalp |
40 ms |
Somatosensory cortex |
Clinical uses
Spinal surgery: Changes in latency and amplitude can be monitored during positional manipulations, including open or closed reduction of spinal deformities. Extradural manipulations, including surgery on disk or vertebral segments, or on epidural abscess or neoplasm, can be monitored with SSEP. Resection of intradural and intramedullary lesions, including tumors and arteriovenous malformations, also can be monitored. Recognizing that SSEP recording monitors primarily the integrity of dorsal columns is important. Inability to test motor pathways, which probably are more important clinically than dorsal column integrity, is a significant limitation of the technique.
Cranial/vascular surgery
-
Carotid surgery including endarterectomy: Changes in SSEP recordings are sensitive for detection of cerebral ischemia. SSEP monitoring can be helpful in determining the need for shunting during the surgical procedure.
-
Cerebral aneurysm surgery: Changes may indicate occlusion of parent vessel branches, which potentially could be reversed by repositioning of aneurysm clips. SSEP monitoring can signal changes prior to irreversible cerebral ischemia. Amplitude and latency of the N20 peak, central conduction time (CCT), and latency difference between the N14 and N20 peaks are reliable indicators of cerebral hemispheric function in aneurysm surgery. [8]
-
Aortic cross-clamping: Changes in SSEP indicate a high risk of neurological injury, especially if the changes are immediate.
Localization of sensorimotor cortex: Localization of the motor cortex is important to minimize the risk of contralateral motor deficits resulting from surgical procedures in its vicinity. The location of primary motor cortex varies to a high degree. Direct cortical stimulation is one technique of localization, though it has limitations. When recording SSEP, the primary sensory cortex and motor cortex generate potentials that are mirror images of each other. This "phase reversal" across the central sulcus is a highly reproducible characteristic that can aid in the localization of primary motor cortex.
Brainstem Auditory Evoked Potentials
Brainstem auditory evoked potentials (BAEP) record cortical responses to auditory stimuli. This allows monitoring of the function of the entire auditory pathway including acoustic nerve, brain stem, and cerebral cortex. Other terms for this technique include brainstem auditory evoked responses (BAER), auditory evoked potentials (AEP), and auditory brainstem responses (ABR).
Technique
Recordings are obtained by stimulating with auditory clicks in the ear. Standard EEG cortical montage is used with recordings obtained from scalp electrodes. Best responses are obtained from electrodes near the ears (A1, A2) referenced to the vertex (Cz). Auditory clicks are delivered in a repetitive pattern, often at 11 Hz, with a frequency that does not coincide with the 60-Hz noise of electrical AC current.
Interpretation
Positive deflections are termed waves I-VII. Waves I, III, and V are the waves most consistently seen in healthy subjects (obligate waves). Wave V is the most reliably seen wave, particularly in patients with hearing impairment or undergoing surgery. A shift in latency of 1 millisecond or a drop in amplitude of 50% could be significant and should be reported to the surgeon.
Table 2. Interpretation of BAEP Waves (Open Table in a new window)
Name |
Probable Anatomical Location |
P1 (wave I) |
Action potential of distal acoustic nerve |
P2 (wave II) |
Proximal acoustic nerve/cochlear nucleus |
P3 (wave III) |
Lower pons |
P4 (wave IV) |
Mid/upper pons |
P5 (wave V) |
Lower midbrain |
Clinical uses
Cerebellopontine angle surgery: This includes surgery for acoustic neuroma or meningioma, or for microvascular decompression for tic douloureux or hemifacial spasm. Important parameters to monitor include peak amplitude of waves III and V, latency of wave V, latency of waves I-V, and latency of waves I-III. If changes occur, they may be due to improper retraction on the cerebellum and brain stem; these may be reversible with a change of position of the retractors by the surgeon.
Motor Evoked Potentials
SSEP has been the standard of intraoperative monitoring, with excellent ability to assess dorsal column and lateral sensory tract function; it probably also can detect changes in function of anterior motor tracts by stimulating mixed sensorimotor peripheral nerves. However, significant motor deficits have been seen in patients undergoing spinal surgery despite normal SSEPs. MEPs were developed to better the motor neurophysiological pathways. Note that anesthetic agents can severely diminish the motor evoked responses.
Technique
MEPs are elicited by either electrical or magnetic stimulation of the motor cortex or the spinal cord (see the image below). Recordings are obtained either as neurogenic potentials in the distal spinal cord or peripheral nerve, or as myogenic potentials from the innervated muscle. Transcranial electrical stimulation involves stimulation of electrodes on the scalp, or if the brain is exposed by a craniotomy, stimulation of electrodes placed directly on the brain surface. [7]
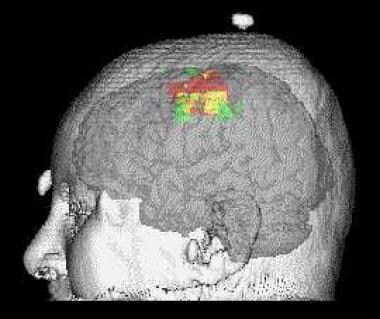
Electrical stimulation also can be applied directly over the spinal cord when a laminectomy affords exposure proximal to the lesion in question. Distal neurogenic potentials then can be recorded.
Transcortical magnetic stimulation delivers a pulsed magnetic field over the scalp in the region of the primary motor cortex. The basis for electrical stimulation generated by applying a magnetic field is based on the Faraday law, which states that a changing magnetic field induces an electric current in a nearby conductor. Unfortunately, generating good signals in the operating room with this technique is difficult; also, the devices necessary to apply strong magnetic fields can be a hindrance in surgery.
MEPs can be obtained reliably and are useful for monitoring of motor pathway function if performed by experienced neurophysiologists. Transcranial electrical motor evoked potentials (TceMEP) have been used more frequently in spinal surgery. Its use in combination with SSEP appears to improve the accuracy of monitoring spinal cord function.
Visual Evoked Potentials
Monitoring of visual pathways has potential utility in surgery performed in proximity to the visual apparatus, especially in the parasellar region. Tumors that arise in this area include craniopharyngiomas, pituitary adenomas, and suprasellar meningiomas. Resection of these tumors carries significant risk of visual impairment.
VEPs have been performed successfully to aid in the monitoring of visual function during surgery in the hope of detecting visual impairment before it is irreversible. It has potential usefulness in assessing integrity of visual pathway structures including optic nerves; however, it cannot detect the presence of visual field defects. Intraoperative use of this technique is still in its infancy; further work is required to determine its full clinical utility.
Technique
Visual stimulation is given by flashing light-emitting diodes (LED) or strobe lights. Potentials are recorded with scalp electrodes. Signal-averaging and noise-reduction techniques are used. Cortically recorded monitoring has also been advocated. [9]
Interpretation
Typically 3 negative peaks (N1, N2, N3) and 3 positive peaks (P1, P2, P3) are seen. The P1-N2-P2 complex typically is monitored during surgery. Latency and amplitude changes are recorded.
Clinical uses
Experiences described in the literature regarding the clinical utility of intraoperative VEP have been conflicting. Monitoring has been performed in tumor resections that require manipulation of the optic apparatus, but its use has not yet become standard practice. Cortically recorded monitoring may provide better assessment of posterior visual pathways. [9]
Effectiveness of Intraoperative Evoked Potential Monitoring
Studies have shown the effectiveness of intraoperative monitoring in improving surgical outcome. In a large study, Nuwer et al showed a 60% reduction of complications in scoliosis surgery by the use of somatosensory evoked potentials. Motor evoked potential monitoring, which can be superior in detecting motor deficits, is less commonly used but does appear to also confer a significant benefit when monitored intraoperatively. Combining SSEP and MEP monitoring modalities seem to improve detection of significant intraoperative changes. [10]
Electroencephalography And Electrocorticography
Electroencephalography
Technique
Intraoperative scalp EEG recordings can be performed using standard electrodes and paper or digital EEG machines. Because of the difficulty of re-applying electrodes in the operating room, a secure scalp-electrode interface must be assured, usually by using collodion with a cup electrode. In certain circumstances, an electrode cap or needle electrodes may be useful. Standard 10-20 electrode placement is used typically, and signals are recorded in 8-32 channels of bipolar, with or without referential, derivations.
Because of the need to monitor beta activity, high-frequency filters less than 35 Hz should not be used, and 70 Hz is preferable; a 60-Hz notch filter is usually necessary. Low-frequency filtering of 1 Hz is usually adequate, along with standard sensitivities of 5, 7, or 10 µV/mm. A paper speed slower than the standard 30 mm/s is often useful for showing asymmetric slowing without obscuring asymmetries of fast activity. Single-channel ECG recording can clarify the source of pulse, ECG, and ballistocardiogram artifacts.
Computerized techniques, such as compressed spectral array, can offer a more visually transparent display of EEG activity, and sometimes aid in interpretation, but for the experienced technologist or electroencephalographer, standard recording can show changes more quickly.
Interpretation
The most important requirement for intraoperative EEG recording is knowledge of expected changes with deepening levels of anesthesia. Premedication with barbiturates or benzodiazepines causes increased beta activity and then successively increased slowing. With induction, frontal intermittent delta activity (FIRDA) often is observed, or perhaps transient (< 1 min) burst suppression if induction is rapid. Then diffuse faster activity is seen, typically slowing from beta to alpha frequencies, superimposed on variable theta and delta, depending on depth of anesthesia. Still deeper stages sometimes can produce burst suppression.
Intraoperative EEG changes can be diffuse, lateralized, or localized. Diffuse changes result mainly from anesthesia changes, as above. Any changes that cannot be explained in this way may reflect changes in systemic blood pressure and/or cerebral blood flow and should be reported to the anesthesiologist and surgeon. The most important lateralized or localized changes include loss of fast activity along with increase in slow activity. At times, fast activity can decrease without an increase in slow activity; the opposite rarely occurs. These lateralized or localized changes generally reflect focal decrease in cerebral blood flow resulting from either acute change in vessel caliber or hypotension in the setting of a fixed stenosis.
Clinical uses
The most common use of scalp EEG for intraoperative monitoring is during carotid endarterectomy. EEG changes are reliable guides to acute changes in cerebral blood flow that occur, for example, during carotid cross-clamping. These usually are seen within 30 seconds and indicate a need for shunting. Embolization during or after the procedure also can manifest as lateralized or localized EEG changes.
Other uses of scalp EEG include during aneurysm repair when carotid clamping is required and during hypothermic circulatory arrest for cardiac surgery.
Electrocorticography
Technique
Intraoperative ECoG can be recorded by using saline-soaked cotton or carbon ball electrodes attached by flexible wires to a frame fixed to the skull, or by using stainless steel or platinum disc electrodes embedded in silastic, similar to those used routinely for long-term extraoperative recording. The signal is recorded with a standard paper or digital EEG machine and displayed in bipolar and/or referential derivations. In the latter case, the reference can be extracerebral, such as a dural needle or skin electrode, or intracerebral, taking care to use a contact well away from the area of interest. Optimal sensitivity settings usually range between 20 and 70 µV/mm, with a high-frequency filter of 70 Hz, 60-Hz notch filter on if necessary, and low-frequency filter of 1 or occasionally 3 Hz. Standard paper speed of 30 mm/s is preferred.
Interpretation
Epileptiform spikes are significantly sharper at the cortical surface than at the scalp and often have durations of only 10-20 milliseconds. Discharges of longer duration and less sharpness likely are generated at a distance and are of questionable significance. The implications of even definite spikes are controversial if they occur only sporadically, while cortical regions producing frequent spikes, occurring periodically to continuously, almost certainly need to be resected for optimal seizure outcome.
As noted later, deep anesthesia with most agents suppresses spikes, while methohexital boluses of up to 1 mg/kg can at times be activating. In awake patients, encouragement to relax and become drowsy can be a more physiologic activation method.
Clinical uses
ECoG is used in a variety of situations in which a goal of surgery is to ameliorate seizures. In lesional cases, seizure outcome is most dependent on complete lesion resection, but removing surrounding areas that show very frequent spiking probably can improve outcome further and also may help if the lesion cannot be removed completely. In the special case of cortical dysplastic lesions, ictal or near-ictal ECoG patterns are common, and may guide resection when the margins are not clear on direct inspection or on neuroimaging.
Nonlesional cases usually rely on extraoperative recording of seizure onsets using indwelling depth or subdural electrodes, but margins sometimes can be refined using intraoperative ECoG. In the case of mesial temporal lobe epilepsy, ECoG recorded from the lateral temporal cortex is of questionable utility, but recording from the parahippocampal region can assist in determining the posterior resection margin.
Cortical Electrical Stimulation
Technique
"Mapping" of functional cortex by electrical stimulation still is considered the criterion standard of determining areas whose resection risks causing neurologic deficits. Usually bipolar stimulation is performed with either the same silastic embedded disc electrodes used for recording, or a movable, hand-held bipolar stimulator including 2 closely spaced spherical electrodes. The stimulus is an alternating square-wave pulse of 0.3-2 milliseconds duration at 50-75 Hz, with currents between 0.5-15 milliampere applied for 4-8 seconds.
In the lightly anesthetized patient, motor cortex can be localized, but the patient must be awake for testing sensation, language, and at times memory formation. Motor-inhibitory areas also can be localized by using continuous motor tasks. Language tasks can include spontaneous speech, recitation, reading, and naming.
Interpretation
Any stimulated area that produces spontaneous movement or sensation should be spared resection if possible. Resection of motor-inhibitory areas also should be minimized, although the deficits produced are usually temporary. Language problems can be minimized by avoiding resection of areas within 1 cm of those producing deficits on any language task; notably, these areas often can be quite removed from the classic Wernicke and Broca areas.
Clinical uses
Cortical electrical stimulation should be considered whenever resection in the vicinity of eloquent cortex is planned. It is used in conjunction with removal of neoplastic, vascular, or other lesions, or of structurally intact cortex for relief of epilepsy.
Effects Of Anesthetics On Evoked Potentials And EEG
Anesthetics exert their effects on the brain by depressing cerebral metabolism. This results in alteration of EEG recordings of the brain. Each type of anesthetic agent alters the evoked response in different ways.
Volatile anesthetics
The volatile agents, which include the halogenated anesthetics and nitrous oxide, produce a dose-dependent depression of cerebral metabolism. They have the most potentially deleterious effect of all anesthetics. All cause similar depression of evoked potentials and prolongation of latencies. They affect cortically evoked responses more than subcortical, spinal, or peripherally evoked responses. At high concentrations, most also can suppress epileptiform discharges.
Barbiturates
These may decrease evoked potential amplitude and lengthen latency, but typically recordings can be obtained despite high doses. They also increase beta frequency activity. Fast-acting barbiturates (eg, methohexital) sometimes can increase epileptiform spikes.
Etomidate
In low doses, etomidate can increase evoked potential amplitude but prolong latencies. At induction doses, amplitude may be reduced.
Ketamine
Ketamine either does not affect or may increase evoked potential amplitude.
Narcotics
Narcotics cause mild reduction in amplitude of evoked potentials but usually allow consistent monitoring.
Benzodiazepines
Benzodiazepines usually result in decreased amplitude with little effect on latencies. Like barbiturates, they increase beta activity (more over normally than abnormally functioning cortex), but they typically decrease rather than increase epileptiform activity.
Neuromuscular blockers
These agents have no significant effect on evoked potentials. Muscle relaxation reduces artifactual signals from spontaneous muscle activity and, if complete, suppresses evoked muscular responses as well.
-
Normal brainstem auditory evoked potentials (BAEP).
-
Normal baseline SSEPs. Left median on upper left corner, right median on upper right corner, left tibial on lower left corner and right tibial on lower right corner.
-
Example of motor map obtained by transcranial magnetic stimulation using a stereotactic technique. The TMS map is represented in red. It is compared to the map that is obtained with functional MRI in green. The overlap of TMS and functional MRI maps is represented in yellow. The 2 techniques can provide complementary information on motor control.
-
Photomicrograph showing differences between the most commonly used needle electrodes: monopolar (MON) and concentric (CN). Courtesy of www.casaengineering.com.