Overview
Maternal alloimmunization, also known as isoimmunization, occurs when a woman's immune system is sensitized to foreign erythrocyte surface antigens, stimulating the production of immunoglobulin G (IgG) antibodies. [1]
The most common routes of maternal sensitization are via blood transfusion or fetomaternal hemorrhage (ie, transplacental passage of fetal erythrocytes) associated with delivery, trauma, spontaneous or induced abortion, ectopic pregnancy, or invasive obstetric procedures. Two studies found that intravenous drug abuse is also associated with alloimmunization. [2, 3] These antibodies can cross the placenta during pregnancies in alloimmunized women and, if the fetus is positive for these specific erythrocyte surface antigens, result in hemolysis of fetal erythrocytes and anemia. This, in turn, can lead to potentially disastrous consequences for the fetus, such as hydrops fetalis (seen below), a high-output cardiac failure syndrome. [4]
With the institution of antenatal Rhesus (Rh) D immunoglobulin prophylaxis, the frequency of maternal alloimmunization in Rh D–negative women has decreased significantly. The American College of Obstetricians and Gynecologists (ACOG) recommends administering Rhesus immunoglobulin to Rh-negative patients with any event in pregnancy that increases risk of fetomaternal hemorrhage and at 28 weeks' gestation. Pending fetal blood type, the patient should also receive Rhesus immunoglobulin following delivery. [5]
Additionally, advancements in fetal surveillance and treatment have allowed for successful outcomes for most affected fetuses. This article reviews the pathophysiology, diagnosis, and management of erythrocyte Rh D alloimmunization and includes a discussion of rarer erythrocyte antigens.
Background
Among the more than 50 different antigens capable of causing maternal alloimmunization and fetal hemolytic disease, the Rhesus (Rh) blood group system is the most common. The Rh blood system is comprised of the c, C, D, e, and E antigens. Of note, no d (pronounced "little d") antigen exists. The D (pronounced "big D") antigen of the Rh blood group system (Rh D) causes most cases of severe hemolytic disease. The incidence of fetuses at risk for anemia due to maternal alloimmunization to red cell antigens has decreased dramatically since the institution of routine anti-D immune globulin (RhoGAM) prophylaxis for Rh-negative women in the 1960s. A review of birth certificate data in 2003 reported the incidence of Rh sensitization to be approximately 6.8 per 1000 live births. [6]
The Rh system was discovered in 1940 when Landsteiner and Weiner performed their landmark experiments wherein rhesus monkey erythrocytes were injected into rabbits and guinea pigs. The resultant antisera were mixed with blood samples from white individuals, and agglutination was observed in 85% of the samples (Rh D positive). The remaining 15% of the samples were Rh D negative; this finding corresponds remarkably to the currently known prevalence of Rh D–negative blood type in whites.
Following this discovery, Levine determined that hemolytic disease of the fetus and newborn was usually caused by Rh incompatibility (ie, the fetal stimulation of Rh D antibody development in an Rh D–negative woman). In 1953, Chown verified that the passage of fetal Rh D–positive erythrocytes into the maternal circulation caused Rh immunization. In 1960, the administration of Rh D IgG, also known as anti-D immune globulin, or RhoGAM, was demonstrated to prevent Rh D alloimmunization, leading to the licensing of anti-D IgG in 1968 for use in North America. Anti-D IgG administration has been enormously successful in preventing maternal alloimmunization.
Pathophysiology
Fetomaternal hemorrhages have been demonstrated to occur in as many as 75% of pregnancies, with the frequency increasing as gestation advances and with most cases occurring during delivery. If transplacental passage of fetal erythrocytes is suspected or at time of delivery, the rosette screening test is used to determine the presence of a fetomaternal hemorrhage. Rosette screening tests are performed by incubating a maternal blood sample with Rh immunoglobulin and enzyme treated red blood cells that make the test results more easily visible. If there are Rh positive red cells in the maternal blood sample, they will be surrounded by the immunoglobulin and indicator cells, forming something that looks like a rosette when observed under a microscope. When the screening Rosette test is positive, the Kleihauer-Betke test is used to quantify the volume of hemorrhage so that an appropriate dose of anti-D IgG can be administered. In many centers, flow cytometry is now used in lieu of Kleihauer Betke testing to quantify fetomaternal hemorrhage as it is automated and more accurate.Hemorrhage volumes sufficient to cause alloimmunization are produced in 15-50% of births. This volume of fetal blood, which, in more than 50% of intrapartum cases can be as small as 0.1 mL and in rare cases can exceed 30 mL, varies depending on the degree of maternal immune response.
As many as 30% of Rh D–negative individuals have been shown to not become alloimmunized, even when challenged with large volumes of Rh D–positive blood. ABO blood group status also affects the risk of alloimmunization. With an ABO-compatible fetus, the overall risk of alloimmunization if not treated with anti-D IgG is approximately 16%. However, in an ABO-incompatible fetus, the risk is only 1.5-2%. The protective effect conferred by ABO incompatibility is believed to be due to maternal destruction and subsequent clearance of the ABO-incompatible fetal erythrocytes before Rh sensitization can occur.
Approximately 17% of Rh D–negative women who deliver an Rh D–positive fetus become alloimmunized if anti-D IgG is not administered appropriately. Of note, because anti-D IgG prophylaxis has reduced the risk of sensitization to less than 1% of susceptible pregnancies, other alloantibodies are now responsible for an increased proportion of hemolytic disease of the fetus and newborn. These include antibodies to other antigens of the Rh blood group system (ie, c, C, e, E) and other atypical antibodies known to cause severe anemia, such as anti-Kell (ie, K, k), anti-Duffy (ie, Fya), and anti-Kidd (ie, Jka, Jkb). (See Atypical erythrocyte antibodies.)
Despite the dramatic success of anti-D IgG prophylaxis protocols, prevention is not universal and 0.27% of susceptible women still become Rh D alloimmunized. One reason for this is failure to follow recommended protocols (see Pregnancy in the nonalloimmunized Rh D–negative patient). Furthermore, a 0.1-0.2% rate of spontaneous immunization occurs despite prophylaxis. These cases have been observed in pregnancies in which no prior overt sensitizing events have occurred. Finally, alloimmunization involving atypical blood groups (eg, Kell and c blood groups) is not yet preventable. Therefore, understanding and using available predictive measures and treatment modalities for hemolytic disease of the fetus and newborn is essential, as is ensuring that the Rh-alloimmunized pregnancy is properly managed.
The results of an epidemiologic study by Pegoraro et al, which estimated the worldwide number of pregnancies involving an Rh(D)-negative mother and an Rh(D)-positive fetus, suggested that about 50% of women around the globe who need immunoprophylaxis do not receive it. [7]
Causes of maternal alloimmunization
Causes of maternal alloimmunization include the following:
-
Blood transfusion
-
IV drug abuse
-
Fetomaternal hemorrhage (antepartum, intrapartum)
-
Abortion (therapeutic, spontaneous, molar pregnancy)
-
Ectopic pregnancy
-
Placental abruption
-
Abdominal trauma
-
Obstetric procedures (eg, amniocentesis, chorionic villus sampling [CVS], percutaneous umbilical blood sampling, external cephalic version, manual removal of the placenta)
Hemolytic disease of the fetus and newborn (HDFN)
IgG antibody–mediated hemolysis of fetal erythrocytes, known as hemolytic disease of the fetus and newborn (HDFN), varies in severity and can have a variety of manifestations. The degree of anemia can range from mild to severe with associated hyperbilirubinemia and jaundice. In severe cases, hemolysis may lead to extramedullary hematopoiesis and reticuloendothelial clearance of fetal erythrocytes. This may result in hepatosplenomegaly; decreased liver function; and ensuing hypoproteinemia, ascites, and anasarca. When accompanied by high-output cardiac failure and pericardial effusion, this condition is known as hydrops fetalis. Without intervention, this syndrome is often fatal. Intensive neonatal care, including emergent exchange transfusion, is required.
Cases of hemolysis in the newborn that do not result in fetal hydrops can still lead to kernicterus. This is a neurologic condition observed in infants with severe hyperbilirubinemia and is due to the deposition of unconjugated bilirubin in the brain. The absence of placental clearance and immature fetal bilirubin-conjugating ability can lead to symptoms that manifest several days after delivery and include poor feeding, inactivity, loss of the Moro reflex, a bulging fontanelle, and seizures. The 10% of infants who survive may develop spastic choreoathetosis, deafness, and intellectual disability.
Various predictive measures are currently available to estimate the severity of fetal disease (see Fetal assessment in the alloimmunized pregnancy). Briefly, these include amniotic fluid spectrophotometric measurements of bilirubin to estimate the degree of hemolysis and anemia, perinatal ultrasonography to assess fetal well-being and to identify findings consistent with hydrops (eg, edema, ascites, effusions), fetal blood sampling ([FBS] also known as percutaneous umbilical blood sampling [PUBS], cordocentesis, and funipuncture) to measure fetal hematocrit directly, and Doppler ultrasonography to measure the peak systolic velocity of blood flow in the fetal middle cerebral artery as an index of anemia.
In utero therapy for fetal anemia has led to reported overall survival rates of 84%, with survival being markedly greater in nonhydropic fetuses. Intrauterine fetal transfusion (IUT) was first introduced by Liley in 1963 via intraperitoneal administration of Rh-negative blood. Advances in ultrasonography led to the development of direct intravascular transfusion, which was first performed in 1982 and has now essentially replaced intraperitoneal transfusion in most centers. A systematic review by de Winter et al found that among pregnancies with Rh(D)-mediated HDFN treated with IUT, the rate of hydrops fetalis was 14.8%. [4]
Range of hemolytic disease of the fetus and newborn (HDFN) is as follows:
-
Hyperbilirubinemia
-
Jaundice
-
Kernicterus
-
Ascites
-
Anasarca
-
High-output cardiac failure
-
Pericardial effusion
-
Hydrops fetalis
Epidemiology
The prevalence of the Rh D–negative blood type is dependent on ethnicity with Whites having the highest prevalence and Asians and American Indians having the lowest.
Rates of Rh D negativity among ethnic and racial groups are as follows:
-
White - 15-16%
-
African American - 8%
-
African - 4%
-
Basque (region of Spain/France) - 30-35%
-
Asian - Less than 1%
-
Asian American - 1%
-
American Indian/Inuit - 1-2%
-
Eurasian - 2-4%
Of the existing Rh antigens, the D antigen is the most immunogenic. Three pairs of Rh antigens are known, with varying gene frequencies and possible combinations. Interestingly, with respect to the c and e alleles of the Rh locus, both c and C as well as e and E antigens exist. With respect to the D allele, d actually refers to the absence of the D allele, as no d antigen is known to exist. When referring to the different alleles, the terms little and big are used. For example, a patient with the cDE complex would be considered to have little c, big d, and big e antigens.
In a retrospective exposure cohort study comprising pregnant women with red blood cell antibodies, those with multiple red blood cell antibodies were more likely (3.65-fold) to develop significant hemolytic disease of the fetus and newborn than those with a single antibody, especially in the presence of anti-(Rh)D. [8, 9]
Approximately 10% of pregnancies in White women are Rh incompatible. However, because the risk of alloimmunization in a susceptible Rh D–negative woman is significantly affected by several factors, less than 20% of Rh D–incompatible pregnancies actually lead to maternal alloimmunization. These factors include the volume of fetomaternal hemorrhage, the degree of maternal immune response, concurrent ABO incompatibility, and fetal homozygosity versus heterozygosity for the D antigen.
Two genes have been identified on the short arm of chromosome 1 that encode the major Rh antigen groups (c/C, D, e/E): RHD and RHCE, with differential protein production in the latter likely due to alternative splicing. Inheritance of these so-called Rh locus genes is closely linked, thus allowing estimations of paternal heterozygosity at the Rh D locus to be made using gene frequency tables that incorporate data on paternal ethnicity, blood type, and number of previous Rh D–positive infants. For example, a White male with blood type CDe who has fathered no prior Rh D–positive children has a 9% chance of being heterozygous, whereas the same man with the blood type cDe has a 94% chance of being heterozygous. As discussed in Assessing fetal risk for Rh D–positive status, paternal genotype (homozygous D/D or heterozygous D/D-, recall that no d antigen exists) contributes to the fetal genotype and phenotype (Rh D positive versus Rh D negative).
Diagnosis
Maternal alloimmunization
Every pregnant woman should have her ABO blood group, Rh type, and antibody screen (indirect Coombs test) checked at the first prenatal visit of each pregnancy. Women found to be D antigen negative with a concurrent negative antibody screen are not alloimmunized and thus are candidates for anti-D IgG prophylaxis as indicated by paternal Rh status (see Pregnancy in the nonalloimmunized Rh D–negative patient). However, if a woman is D antigen negative but with a concurrent positive antibody screen that is not due to iatrogenic administration of anti-D, she is considered alloimmunized and identification of the antibody causing the positive screen, as well as its titer, should be undertaken to guide pregnancy management.
Assessing fetal risk for Rh D–positive status
Determining if the fetus is at risk is important (see the image below) because an Rh D–negative fetus cannot be affected and requires no intervention irrespective of maternal titers. A proposed first step in alloimmunized pregnancies is determining paternal Rh status. With certain paternity, if the father is Rh D negative (genotype D-/D-, which is the absence of D/D since there is no alternative d antigen), the fetus must also Rh D negative, and further follow up surveillance for fetal anemia is not required.
If the father is Rh D positive, he can be either homozygous or heterozygous for the D allele. If he is homozygous for the D allele (ie, D+/D+), then the fetus is Rh D positive. If he is heterogenous (D+/D-), there is a 50% chance that the fetus is also Rh D positive. Further testing is then required to determine fetal Rh phenotype (see section below).
If paternity is not completely certain, or if the father is heterozygous for the D allele, determining fetal D antigen status is the next step. This can be accomplished by polymerase chain reaction assays of fetal cellular elements in amniotic fluid or chorionic villi. Although chorionic villus sampling (CVS) can be performed at earlier gestational ages than amniocentesis, CVS has the disadvantage of potentially worsening maternal titers via fetomaternal hemorrhage that might result from disruption of the chorionic villi during the procedure. Thus, amniocentesis is the recommended method.
In addition to the invasive method of amniocentesis, noninvasive means of determining fetal D antigen status using maternal plasma are now available using cell-free fetal DNA as early as 10 weeks of pregnancy. The first examined clinical application of cffDNA was for determination of fetal RHD status, [10] only later applied to aneuploidy screening. In Europe, other alleles including C, c, E, e, and K can also be tested with cell-free fetal DNA analysis, [11] although currently only testing for RHD is approved for use in the United States. A recent prospective cohort study of 520 non-alloimmunized RhD-negative patients found that in only 0.32% (one case of the 520) was a fetus identified as RHD negative when in fact RHD positive and the authors concluded that it has a high degree of accuracy regardless of the semester when the sample is obtained. [12]
The need for absolute certainty regarding paternity in these settings cannot be overemphasized because the outcomes can be disastrous if the fetus is misdiagnosed as Rh D negative and appropriate fetal surveillance does not occur. Therefore, emphasize this point with patients in a private manner after they have been isolated from anyone who may have accompanied them on their prenatal visit.
Of note, because of the small risk that amniocentesis poses to an at-risk pregnancy, many women choose to obtain fetal Rh status determination only if their anti-Rh titer reaches the critical value of 1:16 or higher. Many women also refuse invasive testing to determine fetal antigen status even with elevated antibody titers. If fetal antigen status cannot be determined, monitoring for development of fetal anemia is performed with MCA Dopplers. The patient should be counseled about the potential consequences of false negative and false positive findings with MCA surveillance which may occasionally lead to cordocentesis in the non-anemic fetus.
A prospective study of 165 alloimmunized pregnancies examined the ability of MCA to accurately diagnose fetal anemia, as defined as hemoglobin at least 5 standard deviations below mean for gestational age. [13] At a peak systolic velocity of 1.5 Multiples of the Median for gestational age or greater, the investigators found that the MCA was only 82% specific. It is not uncommon to perform percutaneous umbilical cord sampling (PUBS) as a result of suspected fetal anemia based on MCA only find a normal fetal hematocrit. PUBS carries an approximate 2% risk of fetal loss. Patients should be counseled that determination of fetal RH status could prevent the possibility of an unnecessary PUBS on fetus with a non-susceptible phenotype (RHD negative) that could lead to pregnancy loss.
Management of Pregnancy
Pregnancy in the nonalloimmunized Rh D–negative patient
The primary goal of caring for an Rh D-negative pregnant woman who is nonimmunized is prevention of alloimmunization. As already discussed, every patient should have her ABO blood group, Rh type, and antibody screen (indirect Coombs test) checked at the first prenatal visit of each pregnancy. Patients who are found to be Rh D negative with concurrent negative results from antibody screens are candidates for anti-D IgG prophylaxis unless the Rh status of the father of the baby is negative and the paternity certain. In theory, if the Rh D status of the father is known, the patient can be counseled regarding the risk of the fetus having the Rh D antigen; however, because of a 3-5% rate of unknown or false paternity, discussing this issue privately with the patient is of the utmost importance. Anti-D IgG is absolutely contraindicated only in those patients with a documented hypersensitivity to anti-D IgG.
Exogenous administration of IgG to suppress an immune response, as in the case of anti-D IgG prophylaxis, is known as antibody-mediated immune suppression. Although several theories have been proposed to explain the mechanism of action of antibody-mediated immune suppression, the most likely mechanism is via central inhibition, wherein Rh D IgG coats fetal erythrocytes, which are then sequestered in the spleen and lymph nodes. The local increase in antigen-antibody complexes interrupts the commitment of B cells to plasma cell clones, thereby suppressing the primary immune response. Additionally, these antigen-antibody complexes stimulate the release of cytokines by immune effector cells that inhibit the proliferation of antigen-specific B cells.
The standard dosing regimen of anti-D IgG is 300 mcg intramuscularly at both 28 weeks' gestation (range, 26-30 weeks' gestation) and again postpartum within 72 hours of delivery unless the neonate is confirmed to be Rh D negative. [14] The 300-mcg dose was determined in 1963 by Pollack et al following experiments in which male volunteers who received Rh D positive erythrocytes were administered varying doses of anti-D IgG to prevent alloimmunization. In the early days of anti-D IgG prophylaxis, most fetomaternal hemorrhages were known to occur during delivery, and thus, anti-D IgG was administered only in the postpartum period. This continues to be the regimen used in many other countries.
Although this produced a dramatic decrease in the prevalence of alloimmunization, Bowman et al observed that despite this postpartum anti-D IgG prophylaxis, 1-2% of susceptible women continued to become sensitized. [15] They concluded that these women were experiencing fetomaternal hemorrhages prior to delivery, and they conducted experiments in which antepartum doses were added to the prophylaxis regimen. This resulted in a reduction in the number of sensitized women from 1.8% to 0.1% and eventually led to a regimen that includes the additional dose at approximately 28 weeks' gestation, which is used today in the United States.
In settings in which the volume of a fetomaternal hemorrhage can be calculated, 10 mcg of anti-D IgG should be administered for every milliliter of fetal blood in the maternal circulation. Thus, the 300-mcg dose is more than adequate for a typical fetomaternal hemorrhage and covers hemorrhage volumes of up to 30 mL of whole fetal blood. In the less than 1% of cases in which the volume of fetomaternal hemorrhage exceeds 30 mL, using the Kleihauer-Betke test or flow cytometry to quantify the volume of fetomaternal hemorrhage and administering the appropriate amount of anti-D IgG (ie, 10 mcg/mL fetal blood) is necessary.
Following delivery, if the infant is found to be Rh D negative, the postpartum dose may be omitted. However, if any doubt remains concerning whether to administer anti-D IgG, always administer unless contraindicated. If the fetus if found to be Rh positive, administer anti-D IgG and take care to screen for excess fetomaternal hemorrhage, particularly if cesarean delivery or manual removal of the placenta occurred because both increase the risk and volume of fetomaternal hemorrhage.
Because delivery of an Rh D–positive fetus is not the only means by which transplacental passage of fetal blood can occur, anti-D IgG prophylaxis for nonimmunized women who are Rh D–negative is also warranted in the following scenarios: first- and second-trimester bleeding, spontaneous or elective abortion, prior to any invasive procedure (eg, amniocentesis), evidence of subchorionic or retroplacental hematoma on ultrasonography, external cephalic version and intrauterine fetal death. Patients who experience antepartum bleeding or intrauterine fetal death in the third trimester should have a Kleihauer-Betke test or flow cytometry to determine whether more RhoGAM (ie, after the prophylactic dose at 28 weeks' gestation) is necessary. If so, patients can be given 10 mcg anti-D IgG per estimated milliliter of whole fetal blood in the maternal circulation.
Because fetal Rh antigens are present as early as the 30th day after conception, anti-D IgG is indicated with ectopic pregnancy and with therapeutic and spontaneous abortions. The risk of alloimmunization in susceptible women undergoing therapeutic or spontaneous abortion is 4-5% and 1.5-2%, respectively. For pregnancies less than or equal to 12 weeks' gestation, 50 mcg of anti-D IgG (also known as MICRhoGAM) is sometimes administered because the entire blood volume of the fetus is usually less than 5 mL. However, pregnancies exceeding 12 weeks' gestation or pregnancies in which the gestational age is unknown should receive the full 300-mcg dose.
The Society of Family Planning advises against routine Rh testing and administration of Rh immunoglobulin before 12 weeks’ gestation for patients who undergo spontaneous, medication, or uterine aspiration abortion. [1] The World Health Organization also recommends against routine Rh immunoglobulin administration for both medical and surgical abortion at less than 12 weeks’ gestation. [16]
Invasive obstetric procedures, such as CVS and amniocentesis (with respective risks of alloimmunization of at least 14% and 7-15%), also necessitate anti-D IgG prophylaxis at the time of the procedure. While a dose of 50 mcg is adequate for first-trimester procedures, the 300-mcg dose should be used for both second- and third-trimester procedures. Additionally, if amniocentesis is performed within 72 hours of delivery, as is often the case with fetal lung maturity determinations, withholding the postpartum anti-D IgG until the fetal Rh status is established postpartum is possible. However, if delivery is to be delayed for more than 72 hours, anti-D IgG should be administered.
Although not an invasive obstetric procedure, external cephalic version is associated with fetomaternal hemorrhage in 2-6% of cases, irrespective of procedure success. In this situation, administering an additional dose or checking for antibody status from the 28-week gestation prophylactic dose and performing a Kleihauer-Betke or flow cytometry test shortly afterward to check for fetomaternal hemorrhage is reasonable. Provided delivery occurs within 3 weeks of the administration of anti-D IgG, a repeat dose is only necessary in the event of a large fetomaternal hemorrhage at delivery.
Although the above guidelines have been dramatically successful in reducing the prevalence of Rh D alloimmunization since the introduction of anti-D IgG prophylaxis in 1968, improper management of nonimmunized Rh D–negative patients continues to be problematic. Potential errors and oversights that can result in the patient becoming Rh D alloimmunized include failing to type every patient with the potential for transfusion or fetomaternal hemorrhage, not screening for fetomaternal hemorrhage, not administering anti-D IgG when indicated, or administering an inadequate dosage of anti-D IgG.
Fetal assessment in the alloimmunized pregnancy
As discussed above, assessment of fetal Rh D status is critical in determining whether a pregnancy in an alloimmunized woman is at risk for the development of hemolytic disease of the fetus and newborn. Once a fetus is found to be at risk (ie, Rh D positive), the goals of managing the alloimmunized pregnancy are 2-fold. First is the detection of fetal anemia prior to the occurrence of fetal compromise. After detection, the goal is to minimize fetal morbidity and mortality by correcting this anemia until fetal lung maturity and delivery can be achieved. Because of the potential need for invasive diagnostic and therapeutic procedures, pregnancies complicated by erythrocyte alloimmunization should be managed by maternal-fetal medicine specialists.
The introduction of measures to both predict the severity of fetal disease and treat it has greatly reduced perinatal mortality rates and augmented survival rates. The decision to use specific interventions should be dictated by the degree of fetal disease. Consider fetal D antigen status, maternal antibody titers, and prior obstetric history to individualize and optimize management of an alloimmunized pregnancy.
First affected pregnancy
Although not reliably accurate in predicting the severity of fetal disease, past obstetric history can be somewhat prognostic. In general, the severity of fetal disease in a particular pregnancy tends to be similar to, if not more severe than, that of prior pregnancies. Additionally, with a history of a prior hydropic fetus, the chance that the next Rh D–incompatible fetus will also become hydropic if untreated is greater than 80%.
In the first affected pregnancy, maternal antibody titer determination is necessary to assess the risk to the fetus and to guide the decision-making process. In general, women with titers higher than 1:4 should be considered Rh alloimmunized. However, the threshold for invasive fetal testing (ie, the critical titer) varies at different institutions and is generally 1:16 or higher because these titers have been associated with fetal hydrops. Titers tend to correlate more reliably with the severity of fetal disease in the first sensitized pregnancy than in subsequent pregnancies. As such, first sensitized pregnancies in which antibody titers are 1:8 or lower can be managed by serially monitoring maternal antibody titers (every 2-4 weeks). If titers remain below the critical titer, delivery can occur at term.
Should titers rise to 1:16 or higher and fetal Rh antigen is D+ or unknown, fetal assessment is indicated via middle cerebral artery peak systolic velocity (MCA-PSV) Doppler ultrasonography or serial amniocentesis for delta OD450 if the former is not available.
History of a previously affected fetus or infant
An anamnestic maternal antibody response typically increases the severity of fetal disease in subsequent pregnancies. In patients with a prior history of a severely affected fetus or neonate, maternal antibody titers are not predictive of the severity of fetal anemia. Instead, fetal assessment for the development of anemia is initiated at 18 weeks.
Ultrasonography
Ultrasonographic evaluation of the fetus is a significant part of managing an Rh-incompatible pregnancy. Accurate determination of gestational age via ultrasonography is critical because management is guided in large part by gestational age. Although it cannot help predict the impending development of hydrops, ultrasonography can help unequivocally diagnose the presence of hydrops—a diagnosis that would greatly affect the course of treatment. The sonographic findings consistent with hydrops include ascites, pleural and pericardial effusions, and edema. See the following images.
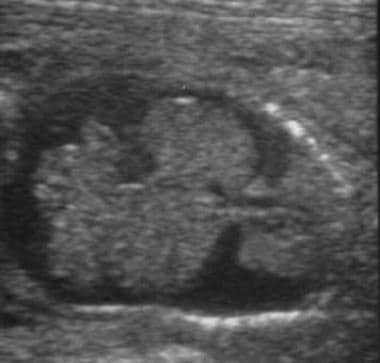
Several other sonographic findings have been proposed as possible indicators of the future development of hydrops. These include polyhydramnios, increased placental thickness (>4 cm), dilation of the cardiac chambers, dilation of the umbilical vein, chronic enlargement of the spleen and liver, and visualization of both sides of the fetal bowel wall. However, none of these findings has proven predictive. Ultrasonography can be used in the management of Rh-incompatible pregnancies to assess fetal well-being; diagnose hydrops; and guide amniocenteses, fetal blood sampling (FBS), and intrauterine transfusions (IUTs). In this capacity, ultrasonography has improved both the safety and success rate of invasive procedures and has helped to minimize invasive testing.
Middle cerebral artery Doppler for MCA-PSV
The use of Doppler flow measurements in various fetal blood vessels has been studied to help predict the severity of fetal anemia. The anemic fetus attempts to enhance oxygenation by increasing cardiac output, thus increasing the velocity of blood flow. Anemia also results in decreased blood viscosity, which in turn results in increased velocity of blood flow. In their studies of the middle cerebral artery (MCA), Mari et al demonstrated that increases in peak velocity of systolic blood flow in the MCA can be used to detect moderate and severe anemia in nonhydropic fetuses. [17] The MCA is used because it can be evaluated using a minimal angle of insonation. Generally, a threshold value of 1.5 multiples of the median (MoMs) is used to determine when FBS with possible IUT should be used. Serial MCA Doppler studies are obtained every 1-2 weeks depending on the trend.
Reliable MCA-PSV values can be obtained as early as 18 weeks' gestation, but care should be taken after 35 weeks' gestation, after which time the false-positive rate of MCA-PSV increases, and consideration should be given to converting to amniocentesis for surveillance and assessment of fetal lung maturity. If MCA Doppler studies are routinely used, an estimated 70% of invasive testing could be avoided.
In a prospective multicenter study of alloimmunized pregnancies and antigen-positive fetuses, MCA-PSV Doppler studies were more sensitive than spectrophotometric analysis of amniotic fluid when using the Liley curve for the prediction of severe fetal anemia. However, MCA-PSV values were not significantly more sensitive when using the Queenan curve. Because amniocentesis is an invasive procedure, MCA Doppler ultrasonography should be preferentially used when available. [18]
Doppler ultrasonography of the MCA had a sensitivity of 88%, specificity of 82% an accuracy of 85%. Comparatively, the amniotic-fluid deltaOD450 had a sensitivity of 76%, a specificity of 77%, and an accuracy of 76%. Based on this, dopplers were more sensitive by 12 percentage points and more accurate by 9 percentage points than the amniotic fluid assessment. [13]
Amniocentesis for delta OD
Prior to the development of MCA-PSV Doppler ultrasonography for fetal assessment, spectrophotometric analysis of amniotic fluid was routinely performed to detect the presence and severity of fetal hemolysis and anemia. Amniotic fluid containing high levels of bilirubin, such as that found in fetuses with severe hemolytic disease, is yellowish-brown. This observation led to the eventual development by Liley in 1961 of a method to predict the severity of fetal hemolysis using spectrophotometric measurements of bilirubin in amniotic fluid. Because the wavelength at which bilirubin absorbs light is 420-460 nm, the amount of shift in optical density from linearity at 450 nm (delta OD450) in amniotic fluid samples can be used to estimate the degree of fetal hemolysis. The Queenan curve (see the image below) is a modification of the Liley curve to adjust for the relative inaccuracy of delta OD450 readings in the early-to-middle secondtrimester.IncontrasttotheLileycurve,whichhas3zonesoffetal anemia, the Queenan curve has 4 zones and has been shown to be more predictive for fetal anemia compared with the Liley curve.
Traditional management of alloimmunized patients with serial amniocenteses (as depicted below) was based on which zone the delta OD450 measurement falls into on the Liley or Queenan curves. Evidence from several studies, including Liley's original work, indicates that mild or no hemolytic disease occurs in zone 1; intermediate disease occurs in zone 2 (transitional between mild and severe hemolysis); and severe disease, including the development of hydrops within the week, occurs in zone 3.
Based on this evidence, once serial measurements are started, if a zone 1 reading is obtained, monitoring the delta OD450 approximately every 3 weeks is reasonable. However, with a trend into zone 2, the frequency of testing should increase to every 1-2 weeks depending on the steepness of the slope of the curve and the closeness of the measurement to zone 3.
Fetal blood sampling
The previously discussed predictive measures are indirect measures of fetal disease. The only definitive means of diagnosing fetal anemia and acidosis is via fetal blood sampling (FBS), also known as cordocentesis or percutaneous umbilical blood sampling (PUBS), which was first performed in the early 1980s. FBS helps provide direct and accurate diagnosis of anemia and fetal acidosis.
The other advantage of FBS is that by providing direct access to the umbilical vein, the same procedure can be used to transfuse the fetus if indicated by fetal hematocrits less than 30%. Despite the wealth of information afforded by FBS, routine use for screening for fetal anemia is not universal because of concerns regarding fetal and maternal complications. These include fetomaternal hemorrhage, fetal loss (0.5-2%per procedure), placental abruption, acute refractory fetal distress, and amnionitis with maternal adult respiratory distress syndrome.
Intrauterine transfusions
Once the MCA Doppler peak systolic velocity reaches more than 1.5 MOM for gestational age (or the delta OD450 measurements have entered high zone 2 or zone 3, or the fetus has been diagnosed with hydrops based on ultrasonographic findings), a decision should be made to perform an FBS with possible IUT if the hematocrit is less than 30%. Alternatively, if beyond 34 weeks' gestation, the decision is to proceed towards delivery (32 wk with mature lung indices). Numerous important factors must be considered when preparing a patient for FBS and possible IUT, including gestational age, the possibility of delivery, fetal maturation with corticosteroids, and the likelihood of transfusion.
If a patient is beyond viability (24 wk), a discussion regarding the management of fetal bradycardia should occur. Even though 24 weeks' gestation is considered the cusp of viability, the outcomes are likely to be even more dismal if the fetus is hydropic. In this setting, consultation with a neonatologist is important, as is fully informing the patient of the description and probabilities of different outcomes.
If the fetus is at a gestational age at which the patient desires immediate delivery if signs of fetal distress occurred, the procedure may be performed with the patient under epidural or spinal anesthesia to avoid the risks associated with general anesthesia and to decrease the amount of time required to deliver the fetus. In addition, also consider regional anesthesia in the setting of likely transfusion because this procedure can be time consuming and uncomfortable for the patient. Because of the possibility of immediate delivery, a 48-hour course of betamethasone to accelerate fetal maturity, particularly the lungs, is usually administered before the first 2 procedures.
The first IUTs were performed intraperitoneally in 1963. However, since the advent of FBS and intravenous transfusions into the umbilical vein, use of the intraperitoneal transfusion (IPT) has diminished in the management of anemic fetuses. Benefits of IVT over IPT include direct measurement of the fetal hemoglobin and acid-base status, lower failure rate (particularly in hydropic fetuses), lower rates of procedure-related morbidity and mortality, and better efficacy at earlier gestational ages. The only benefit offered by IPT is the ability to drain fetal ascites during the procedure, but this is of minimal benefit in hydropic fetuses. IPT may additionally be used in the severely anemic early second trimester fetus (18-24 weeks' gestation) due to technical imitations in accessing the umbilical vein (small caliber).
When preparing to perform an FBS, a number of issues must be addressed before the procedure itself. As mentioned previously, the patient must have a consultation with a neonatologist and anesthesiologist. The blood for possible transfusion must be typed and cross-matched against the mother's serum to help rule out any other possible hemolytic antibodies. Additionally, the unit of red blood cells should be cytomegalovirus-negative, irradiated, less than 5 days old, and with a hematocrit level between 75-80%. This can require several hours. The large variety of equipment, such as transfusion tubing, a blood warmer, heparinized syringes, and an accurate machine to obtain a rapid hematocrit value, must be prepared and calibrated. Unless the procedure is being performed in a unit that routinely performs these procedures, these issues should be discussed and reviewed in detail with the team of nurses, physicians, and technologists involved in the procedure.
The amount to be transfused can be calculated once the hematocrit/hemoglobin results are returned. In general, 30-60 mL/kg of nonhydropic fetal weight is transfused because volumes higher than this may be difficult for the fetus to tolerate. Once the transfusion is accomplished, a final blood sample is often taken to estimate the final hematocrit value of the fetus, although this measurement is an underestimation of the hematocrit secondary to the large volume infused during the transfusion. The fetus should be monitored with continuous fetal monitoring for the ensuing 4 hours (both during the procedure and immediately after because decelerations of the fetal heart rate are common and must be managed cautiously).
If the initial hematocrit value was extremely low, a repeat procedure may be necessary as soon as within a week. Otherwise, the procedure can be performed every 2-4 weeks based on the post-transfusion hemoglobin value. Because the goal is to maintain the fetal hemoglobin value at greater than 9 g/dL and because it drops at approximately 1 g/dL every 3 days, these values can be used to calculate how much time can be allotted until the next procedure. Alternatively, the MCA peak systolic velocity may be used to time the second IUT, typically using a threshold of 1.32 MOM, though this is not well established. Serial IUTs are usually performed until 34 weeks' gestation, beyond which time the risk of the procedure likely outweighs the benefits. This leads to delivery of the fetus at 34-37 weeks' gestation and, occasionally, even earlier if fetal lung maturity is documented.
Antenatal testing
Additional fetal assessment via antenatal testing with nonstress tests or biophysical profiles should begin at 32 week’ gestation in all gestations complicated by erythrocyte alloimmunization.
Timing of delivery
In determining the optimal delivery time, gestational age, severity of fetal anemia, and fetal lung maturity should all be considered. If fetal surveillance remains reassuring (ie, serial MCA-PSV studies remain < 1.5 MoMs or spectrophotometric analysis does not indicate severe fetal anemia), labor should be induced at 37–38 weeks of gestation after confirmation of fetal lung maturity to allow fetal hepatic maturation.
After 35 weeks’ gestation, the rate of false-positive results from MCA-PSV studies is increased. Thus, fetal assessment should be performed via amniocentesis, which has the additional benefit of allowing assessment of fetal lung maturity. Between 36-38 weeks’ gestation, if fetal lung maturity has not been achieved but the fetus is found to be in an upper affected zone, consideration should be given to administration of oral phenobarbital (30 mg tid for 7 d) to accelerate hepatic maturity in an effort to minimize hyperbilirubinemia, and labor should be induced in 1 week. However, many clinicians may opt for immediate delivery at this point to minimize risk from further hemolysis or additional invasive procedures.
Alloimmunization to Non–Rh D Antigens
Although alloimmunization to the Rh D erythrocyte antigen has historically been the most common etiology of severe hemolytic disease of newborns, it is becoming rare in developed countries because of routine prophylaxis with anti-D IgG. However, hemolysis may also be caused by the presence of antibodies to atypical erythrocyte antigens. No screening program or prophylaxis is available for these atypical erythrocyte antigens in antigen-negative patients. Sensitization to these atypical erythrocyte antigens can lead to fetal hemolysis. Consequently, the rate of hemolytic disease related to these more rare antibodies is either stable or rising.
In a Swedish linked national health and data registers study, investigators reported that 1.3% of more than 1 million study pregnancies were alloimmunized and that alloimmunization with Rhesus (c, C, D, E), K- and -Lea red blood cell antibodies increased the risk of preterm birth and/or stillbirth. [19] Moreover, compared with firstborn children, subsequent births were at greater risk of preterm births.
Commonly, patients with antibodies to the rarer erythrocyte antigens are recognized by the antibody screen performed upon initial presentation to prenatal care. If the results of the screen are positive, the laboratory isolates the exact antibody. If the antibody is known to cause hemolysis in the fetus (see Association of atypical erythrocyte antibodies and hemolytic disease of the newborn), such as anti-c, anti-Kell, and anti-E antibodies, these patients are treated similarly to those who are Rh sensitized. Initial management involves monitoring serial maternal antibody titers, which leads to an assessment of the fetus for hemolysis using amniocentesis or MCA Doppler studies as indicated by rising antibody titers, ultrasound findings, and possibly, the results from FBS.
If the antibody has not been known to cause fetal hemolysis (see Association of atypical erythrocyte antibodies and hemolytic disease of the newborn), such as anti-Lea and anti-Leb (the Lewis antigens), no reason exists to follow antibody titers. One proposition is that patients with antibodies known to cause only mild hemolysis, such as anti-Hil, anti-Hu, and anti-Vw antibodies, can be treated expectantly because the risk of invasive testing likely outweighs the risk of disease. In the rare case of finding a new erythrocyte antibody, conservative management entails monitoring the patient as if the antibody would cause hemolysis. However, patients should be counseled carefully regarding the risks and benefits of either management plan.
In only 2 situations are patients not monitored identically to patients who are Rh sensitized. The first is that of alloimmunization to the c, E, or, C antigens. Some concern exists that hemolysis may occur in these patients with a lower than 1:16 titer. Thus, if the initial titer is 1:4 and stable but increases at 26 weeks' gestation to 1:8, assessment with MCA Doppler velocity at that point is reasonable. However, if the patient presents in the first trimester with a 1:8 titer that remains stable at 1:8 throughout the second trimester, continued serial antibody titers are appropriate.
The second situation in which patients should not be treated identically to patients who are Rh D sensitized is that of Kell isoimmunization because several cases of severe fetal hemolysis with anti-Kell antibodies have occurred in the setting of low titers (ALTHOUGH 1:8 is reasonable) and low delta OD450 values. The proposed etiology for this is that the anti-Kell antibodies may attack and destroy erythroid precursors in contrast to causing hemolysis, in the manner of the Rh D antibody. This leads to fetal anemia, but not with the concomitant rise in bilirubin breakdown products, thus leading to relatively normal values of the delta OD450 and rendering amniotic fluid studies uninformative. MCA Doppler flow studies are thus used in these patients to assess fetal anemia.
Association of atypical erythrocyte antibodies and hemolytic disease of the newborn (with disease frequency and antibodies) is as follows:
-
Common – Kell, c, E [20]
-
Uncommon - e, C, cE, Ce, Cw, Kpa, Kpb, k, Jka, s, Wra, Fya
-
Rare - Biles, Coa, Dia, Dib, Doa, Ena, Fyb, Good, Heibel, Jkb, Lua, Lub, M, Mia, Mta, N, Radin, S, U, Yta, Zd
-
No documented cases - Lea, Leb, P
Conclusion
The prevention and treatment of Rh D alloimmunization leading to fetal hydrops is a true success story in obstetrics. While this problem only occurred in a minority of pregnancies, the outcomes were disastrous in patients who were affected. Discovery of the Rh D antigen and implementation of anti-D IgG prophylaxis to prevent sensitization in 99% of potential cases now allows for good outcomes in Rh D-negative women. Furthermore, in patients who have become sensitized, close monitoring of antibody titers, the use of MCA-PSV or delta OD450 to recognize hemolysis or anemia, and treatment with IUT have led to a dramatic decrease in perinatal morbidity and mortality rates. The development of noninvasive modalities to assess fetal anemia has allowed increasing avoidance of amniocentesis and its associated risks, and it is hoped that with continued research, more noninvasive modalities will be possible in the future.
-
Ultrasound images of hydrops. Transverse image of fetal abdomen with ascites.
-
Hydropic fetus. Ultrasound image of coronal view of fetus with ascites and bilateral pleural effusions.
-
Fetal hydrops. Ultrasound image of scalp edema in a hydropic fetus.
-
Fetal hydrops. Ultrasound image of edema of the scalp and face in a hydropic fetus.
-
Determining fetal Rh D status.
-
Queenan curve. Adapted from Queenan et al, Am J Obstet Gynecol, 1993.