Overview
A physician with bilateral, peripheral vestibular dysfunction described the classic example of the vestibuloocular reflex (VOR) and its functional significance. The physician noticed that he was unable to read street signs while walking. However, if he stood still, objects became clear, and he was able to read again. This description of bilateral, vestibular dysfunction highlights the role of the vestibuloocular reflex in the stabilization of objects on the retina during brief head movements. When walking, vibrations from heel strike are transmitted to the head. A combination of the vestibuloocular reflex and the vestibulocollic reflex (VCR) stabilize visual acuity. Compensatory neck movements mediate the vestibulocollic reflex while head movements mediate the vestibuloocular reflex. Without the stabilizing effect of the vestibuloocular reflex, head perturbations as little as 2 Hz or 80° can result in retinal slip, which significantly alters visual acuity.
Separate studies by Jacobs and Carpenter show that visual acuity declines by 50% at a point 2° from the center of the fovea. [1, 2] The fovea (see the image below) is that part of the retina where photoreceptor density is greatest and visual acuity is highest. Thus, the main purpose of the vestibuloocular reflex is to maintain objects on the fovea, which thereby allows a person to visualize objects clearly during brief head movements.
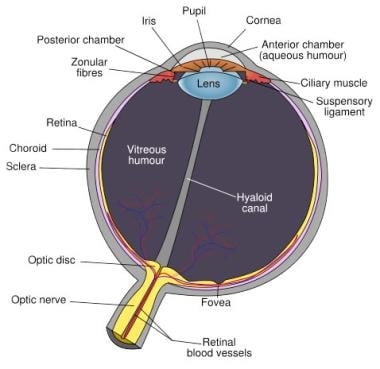
When the head moves, the vestibuloocular reflex responds with an eye movement that is equal in magnitude but opposite in direction (see the image below). Head movements, rotational and translational, stimulate the vestibuloocular reflex. With a rotational movement, the head moves relative to the body. Examples of this include turning the head back and forth, nodding, and bringing the ear in contact with the shoulder. Translational movements occur when the entire body (including the head) is moved in tandem. Translational movements may occur when an individual stands on a moving sidewalk. Thus, rotational vestibuloocular reflex (r-VOR) responds to angular motion of the head and results from stimulation of the semicircular canals, whereas translational vestibuloocular reflex (t-VOR) responds to linear motion of the head and results from stimulation of the otolithic organs. Some head movements may involve a combination of both translational vestibuloocular reflex and rotational vestibuloocular reflex.
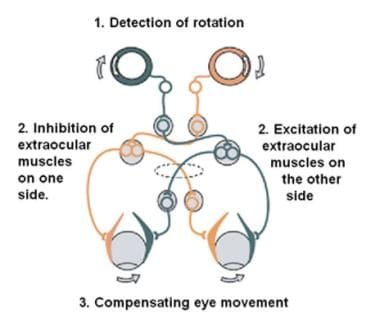
Vestibuloocular reflex anatomy and physiology
A simplistic view of the vestibuloocular reflex (VOR) involves a 3-neuron arc that consists of the vestibular ganglion, vestibular nuclei, and oculomotor nuclei. Although this arc provides a basic framework, the pathways involved in the generation of the vestibuloocular reflex are much more complex.
Rotational vestibuloocular reflex
During rotational movements of the head, the endolymphatic fluid within the semicircular canals shifts because of its inertia, which deflects the cupula. Endolymphatic flow toward the ampulla is excitatory in the horizontal canals, while flow away from the ampulla is excitatory in the superior and posterior canals. Afferent nerves from the ampulla carry both excitatory and inhibitory signals to the 4 major vestibular nuclei: medial vestibular nucleus, lateral vestibular nucleus, inferior or descending vestibular nucleus, and superior vestibular nucleus. Different regions within each of the nuclei project to the oculomotor nuclei (cranial nerves III, IV, and VI). Efferent signals from these nuclei then result in contraction and relaxation of the appropriate ocular muscles.
Excitation of the superior canal results in contraction of the ipsilateral superior rectus and contralateral inferior oblique muscles and relaxation of the ipsilateral inferior rectus and contralateral superior oblique muscles, which results in an upward torsional eye movement. Excitation of the posterior canal results in contraction of the ipsilateral superior oblique and contralateral inferior rectus muscles and relaxation of the ipsilateral inferior oblique and contralateral superior rectus muscles. This results in a downward torsional eye movement. Finally, excitation of the lateral canal results in contraction of the ipsilateral medial rectus and contralateral lateral rectus muscles and relaxation of the contralateral medial rectus and ipsilateral lateral rectus muscles. This results in a horizontal eye movement toward the opposite ear.
The vestibulocerebellum compares input from visual and vestibular sensors and mediates changes in the vestibuloocular reflex after vestibular injury or change in visual function.
In addition to oculomotor projections, the vestibular nuclei send fibers to the vestibulocerebellum, the nucleus prepositus hypoglossi, and the cells within the paramedian tracts. The nucleus prepositus hypoglossi is crucial for the maintenance of a steady gaze, while the cells within the paramedian tracts are responsible for relaying information to the vestibulocerebellum, specifically the flocculus. Reciprocal projections to and from the cerebellum assist in fine motor control of eye movements. The latency of action of the rotational vestibuloocular reflex (r-VOR) is 7-15 milliseconds, which is the time required for the eyes to respond in an equal, but opposite, manner to the motion of the head. This time is remarkably fast compared with the latency for visually mediated eye movements, which is longer than 75 milliseconds.
Finally, recent studies suggest that cerebral function may also be responsible for the modification of the vestibuloocular reflex and the ability to suppress the vestibuloocular reflex. Specifically, injuries to the parietal vestibular cortex and the ocular gyrus appear to interfere with visual suppression of the vestibuloocular reflex. In particular, the right temporoparietal cortex is believed to be involved in the modulation of the VOR. This region has been shown to be sensitive to the effects of sleep deprivation, particularly with respect to VOR gain during step testing. [3, 4]
Translational vestibuloocular reflex
The translational vestibuloocular reflex (t-VOR) pathways are activated in response to stimulation of the otolithic organs. The utricle responds to lateral translation stimuli, whereas the saccule responds to vertical translations. Translational vestibuloocular reflex pathways have not been studied as extensively as the pathways for the rotational vestibuloocular reflex.
However, translational vestibuloocular reflex pathways also appear to be mediated by projections to the ocular motor nuclei via projections from the vestibular nuclei. Specifically, excitation of the utricular macula results in contraction of the ipsilateral superior oblique, superior rectus, and medial rectus muscles and relaxation of the contralateral inferior oblique, inferior rectus, and lateral rectus muscles.
Other vestibular reflexes
Finally, note that the vestibuloocular reflex (VOR) is only one of 3 vestibular reflexes. The vestibulospinal and vestibulocollic reflexes also contribute to stability. The vestibulospinal reflex can be tested with the use of computerized dynamic posturography. The reader is referred to Medscape Reference articles Vestibular Rehabilitation and Inner Ear, Evaluation of Dizziness for additional information.
Of the 3 vestibular reflexes, the vestibulocollic reflex is the least understood. The vestibulocollic reflex is indicative of otolithic (saccular) function and can be elicited by direct (intraoperative) stimulation of the inferior vestibular nerve, acoustic stimuli, mechanical stimulation of the forehead, and galvanic stimulation. The response can be measured as a myogenic potential in the ipsilateral sternocleidomastoid and trapezius muscles. The test used, a vestibular evoked myogenic potential (VEMP) test, continues to involve in its clinical application. For more information on VEMP testing, the reader is referred to Medscape Reference article Inner Ear, Evaluation of Dizziness.
For more information about the relevant anatomy, see Vestibular System Anatomy, Visual System Anatomy, Extraocular Muscle Actions, Extraocular Muscle Anatomy, and Inner Ear Anatomy.
Vestibuloocular reflex dysfunction
Similar to all other systems in the body, most individuals are not aware of the presence of the vestibuloocular reflex (VOR) until it malfunctions. Acute vestibuloocular reflex dysfunction may manifest in several different ways, depending on the anatomical location of the lesion or lesions, and may result from labyrinthine disorders or disorders of the central vestibular system. [5] See the table at the end of this section details.
Studies have shown that patients with a unilateral peripheral vestibular lesion may exhibit asymmetric responses to rotation. On the other hand, patients with a compensated unilateral lesion show a characteristic pattern of decreased gain and increased phase lead at low-frequency stimulation.
Bilateral peripheral vestibular lesions are characterized by low gain and phase lag as determined by sinusoidal testing. These patients commonly report oscillopsia, a sensation of vertical or horizontal motion of the environment, or persistent unsteadiness, especially in the dark. Rotational chair testing is ideal in the assessment of these patients because, unlike caloric testing, higher frequencies are tested and both labyrinths are simultaneously stimulated. This allows for an accurate determination of remaining vestibular function, which is important for determining a course of treatment. [6]
Central vestibular deficits may also affect the vestibuloocular reflex. Thurston et al have shown that gains may be increased in some individuals with cerebellar deficits. Cerebellar atrophy, on the other hand, may result in a disorganized nystagmus pattern with beat-to-beat variabilities in the amplitude. As previously mentioned, lesions within the parietal vestibular cortex and the ocular gyrus may interfere with the ability to suppress vestibuloocular reflex visually.
High-velocity angular vestibuloocular reflex function can also be affected by post-blast exposure, as studied in service members. [7]
Although an impaired VOR is generally the result of an injury to the vestibular system, note that VOR may be affected by systemic disease processes such as migraines, depression, and anxiety disorders. With migraine vestibulopathy, one may see an elevated gain with visually enhanced VOR (VVOR), a testing paradigm where the VOR rotation stimulus is done in a lighted (ie, visually enhanced) environment rather than in the traditional dark booth. Patients who experience anxiety disorders may have an increased vestibular sensitivity resulting in significantly higher VOR gains and shorter time constants. [8] Finally, those patients with major depression have been shown to have hypoactive vestibular nuclei, resulting in a decrease in the slow phase of the nystagmus. All of these disorders should be screened for and considered when testing an individual with vestibular dysfunction.
Table 1. Manifestations of Vestibuloocular Reflex Dysfunction (Open Table in a new window)
Lesion or Disorder |
Clinical Signs and Symptoms |
Gain |
Phase (Time Constant) |
Symmetry |
Unilateral peripheral lesion, acute |
Nystagmus in plane of the affected canal; spontaneous nystagmus |
Decreased toward side of lesion |
Decreased vestibuloocular reflex time constant |
Asymmetric (directional preponderance) |
Unilateral peripheral lesion, compensated |
... |
Still decreased (but closer to 1) |
Time constant rises slightly compared with acute |
Asymmetric |
Bilateral peripheral lesion, acute |
Oscillopsia, visual impairment |
... |
Vestibuloocular reflex time constant < 6 s |
... |
Bilateral peripheral lesion, compensated |
Some oscillopsia with rapid head movement |
Near normal |
Decreased time constant |
... |
Cerebellar |
Oscillopsia, down-beat nystagmus |
Little change (increased or decreased) |
... |
Asymmetric |
Central vestibular dysfunction |
Oscillopsia, purely vertical or torsional nystagmus, balance disorders, spontaneous nystagmus |
Decreased or increased (variable) |
Prolonged or shortened time constant (variable) |
Asymmetric |
Technique
Evaluation of the vestibuloocular reflex
As in the evaluation of all patients, the history and physical examination are the primary determinants of further assessment with laboratory, radiologic, and other ancillary testing. If a patient reports true vertigo, this is suggestive of a dysfunction in the semicircular canals, its central projections, or both. If a patient reports body or room tilt, this implies dysfunction within the otolithic system. Oscillopsia is a symptom reported by patients with vestibuloocular reflex (VOR) dysfunction. Patients with these and other vestibular symptoms often undergo a series of tests for diagnosis and localization of a lesion.
Evaluation of rotational vestibuloocular reflex
The rotational vestibuloocular reflex is analyzed for 3 parameters: gain, phase, and symmetry. Gain is the ratio of the amplitude of eye movement to the amplitude of the head movement (stimulus). Phase is a parameter that describes the timing relationship between head movement and the reflexive eye response. When the head and eyes move at exactly the same velocity in opposite directions, they are said to be completely out of phase, or 180°. If the reflex eye movement leads the head movement, a phase lead is present. Likewise, if the compensatory eye movement trails the head movement, a phase lag is present. Finally, testing is analyzed for symmetry. Symmetry is a comparison of the slow component of the nystagmus when the head is rotated to the right compared with rotation to the left. Each of these parameters is useful in the diagnosis and localization of vestibular lesions.
The simplest way to analyze rotational vestibuloocular reflex is to have a patient read a target such as an index card while the examiner passively rotates the patient's head in a horizontal plane at a frequency of 2 Hz. If the patient's gain is decreased, visual acuity deteriorates and corrective saccades may be noted. However, the patient's visual acuity should not deteriorate with shoulder-to-ear head movements; therefore, this test can be used to help identify patients who are malingering.
A second method of analyzing rotational vestibuloocular reflex gain is with an ophthalmoscope. The patient is asked to view a distant target while shaking his or her head at a frequency of higher than 2 Hz. The examiner then views the optic vessels and disc. Because the eye movement is equal but opposite to the head movement, the vessels and disc should appear stationary. This corresponds to a gain of 1. If the eyes appear to move in the opposite direction of the head, the gain is hypoactive, or less than 1. If the eyes appear to move in the same direction as the head, the gain is hyperactive, or greater than 1.
Although the 2 previously mentioned tests are useful for a quick bedside clinical assessment, the results of both tests are subjective. In addition, phase and symmetry are difficult to assess with these methods. The most commonly used tests for quantifying rotational vestibuloocular reflex abnormalities are caloric testing, rotational chair testing (see the image below), and head autorotation tests. Caloric testing provides information on the horizontal canals at nonphysiologic frequencies and is described in greater detail in Inner Ear, Evaluation of Dizziness. Rotational chair testing provides a detailed analysis of an individual's gain, phase, and symmetry at various rotational frequencies. Rotational chair testing and its interpretation and applications are described in detail in Rotary Chair Testing.
A second method of objectively testing the rotational vestibuloocular reflex is with head autorotation. This technique tests the high-frequency (2-6 Hz) rotational vestibuloocular reflex. One method of testing head autorotation is with standard electrooculography techniques. Electrodes are placed on the face in the standard fashion and are then connected to a computer, which records the impulses from the electrodes. Patients are then asked to rotate their head while focusing on a light, as with other electronystagmography testing. Audible signals that instruct patients to turn their head are given.
Alternatively, head autorotation testing may be conducted with the use of a special headband with rotational sensors. The headband contains an electrooculography amplifier and a calibrated rotational head velocity sensor. This equipment is then connected to a computer, and patients are again presented with auditory cues to rotate their head. The auditory cues start at a frequency of approximately 2 Hz and increase to 6 Hz. One of 2 testing paradigms may be used. Patients may be asked to focus on an object while rotating the head, or their vision may be suppressed with a visor.
The rotational vestibuloocular reflex is analyzed for gain and phase with the use of the head autorotation. Head autorotation with a visor yields results indistinguishable from those of the rotational chair in a dark room. Unlike rotational chair testing, however, autorotation can test rotational vestibuloocular reflex frequencies higher than 1 Hz and can be used for both horizontal and vertical rotational vestibuloocular reflex testing. High-frequency head rotation testing from 2-6 Hz is important because many daily activities involve head movements in these ranges. Head autorotation offers additional advantages over traditional rotational vestibuloocular reflex testing. While providing quantitative data, head autorotation requires less time to run than caloric or rotational chair testing, costs less, and is portable.
Newer methods of testing vestibuloocular reflex continue to be studied. One study has shown that whole-body rotation with the use of a standard swivel chair and passive head rotation also yields results similar to those of the rotational chair, up to a frequency of 1 Hz.
Translational vestibuloocular rotation
The translational vestibuloocular rotation (t-VOR) and interaction of the otolith-ocular responses are not routinely tested in most laboratories. These tests are mostly considered experimental and often involve sophisticated, expensive equipment such as a linear sled cart, off-vertical axis rotation, or a platform suspended from the ceiling (ie, parallel swing).
Newer, less expensive techniques in development include manual translational heaves and a head sled that, unlike the linear sled, moves only the head. Additionally, the clinical significance of translational vestibuloocular reflex continues to be studied. These studies may elucidate the mechanism behind motion sickness and may have a future role in bedside monitoring of patients with unilateral vestibular dysfunction.
Ghoreyshi and Galiana have developed an automated algorithmic tool to analyze VOR responses, which the authors state allows for greater objectivity and, therefore, the design of more complex protocols. [9]
Rehabilitation
Acute vestibuloocular reflex dysfunction, whether peripheral or central, is disturbing to a patient. However, as patients begin to compensate, the dysfunction becomes less debilitating and noticeable in situations that require complex interaction of the 2 systems. Patients can actively promote compensation through a series of rehabilitation exercises.
Vestibuloocular reflex disturbances are treated with different forms of rehabilitation exercises. For example, patients are asked to hold an index card 1 foot from their eyes and to focus on a word or object on the index card as they rotate their head. Refer to Medscape Reference article Vestibular Rehabilitation for more detail. Rehabilitation is adversely affected by vestibulosuppressive medications.
Impact of Age on the Vestibuloocular Reflex
In recent years, studying the natural maturational and aging effects on the vestibuloocular reflex (VOR) have been of particular interest. [10, 11, 12, 13, 14, 15] Studies have shown that the VOR may be present as early as 3 months of age. Furthermore, the absence of VOR by the age of 10 months may be an abnormal finding. Even in premature infants, the VOR is expected to be equivalent to a full term child's by around the age of 9 months.
In one study, children (< 11 y) were shown to have higher gains and phase lead at 0.08 Hz when compared with adults. However, at a higher frequency (0.5 Hz), no difference was found in the data obtained in children relative to adult normative data. A second study also suggests that at higher rotational frequencies (0.5 Hz and 2 Hz), VOR may be mature as early as 8 years of age. These studies show that maturational differences are found in the VOR, and adult normative data may not be appropriate when testing children. [12]
At the other extreme, adult normative data may also be inappropriate when testing the elderly, on whom the natural process of aging may impact the gain of translational VOR. In one study, healthy subjects over the age of 60 were shown to have significantly reduced gains with translational VOR testing when compared with adults younger than 40. [11] This was felt to be the effects of normal aging.
A second study examined the effects of aging on rotational VOR through a 5-year longitudinal study of adults over the age of 75. Healthy subjects were tested annually for 5 years. With sinusoidal rotation, rotational VOR gains and phase leads both increased with age when rotated at a low frequency (0.05 Hz) and high peak velocities (120°/s and 240°/sec). At higher frequencies and lower peak velocities, no significant change was seen. These studies and several others show that the natural maturational and aging processes affect the vestibular system and must be accounted for when testing patients with vestibular symptoms.
-
Rotary chair.
-
The vestibulo-ocular reflex. A rotation of the head is detected, which triggers an inhibitory signal to the extraocular muscles on one side and an excitatory signal to the muscles on the other side. The result is a compensatory movement of the eyes. Image courtesy of Wikimedia Commons.
-
Schematic diagram of the human eye, with the fovea at the bottom. Image courtesy of Wikimedia Commons.