Practice Essentials
Ischemic stroke (see the image below) is characterized by the sudden loss of blood circulation to an area of the brain, resulting in a corresponding loss of neurologic function. Acute ischemic stroke is caused by thrombotic or embolic occlusion of a cerebral artery and is more common than hemorrhagic stroke.
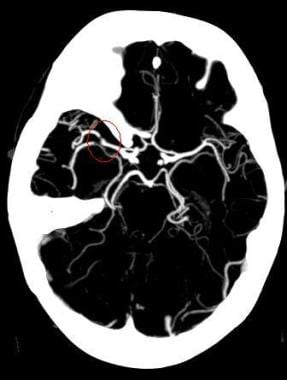
The International Classification of Diseases, 10th Revision, Clinical Modification (ICD-10-CM) code for “cerebral infarction” is I63. It includes occlusion and stenosis of cerebral and precerebral arteries, resulting in cerebral infarction. [1]
Signs and symptoms
Consider stroke in any patient presenting with acute neurologic deficit or any alteration in level of consciousness. Common stroke signs and symptoms include the following:
-
Abrupt onset of hemiparesis, monoparesis, or (rarely) quadriparesis
-
Hemisensory deficits
-
Monocular or binocular visual loss
-
Visual field deficits
-
Diplopia
-
Dysarthria
-
Facial droop
-
Ataxia
-
Vertigo (rarely in isolation)
-
Nystagmus
-
Aphasia
-
Sudden decrease in level of consciousness
Although such symptoms can occur alone, they are more likely to occur in combination. No historical feature distinguishes ischemic from hemorrhagic stroke, although nausea, vomiting, headache, and sudden change in level of consciousness are more common in hemorrhagic strokes. In younger patients, a history of recent trauma, coagulopathies, illicit drug use (especially cocaine), migraines, or use of oral contraceptives should be elicited.
With the availability of reperfusion options (fibrinolytic and endovascular therapies) for acute ischemic stroke in selected patients, the physician must be able to perform a brief but accurate neurologic examination on patients with suspected stroke syndromes. The goals of the neurologic examination include the following:
-
Confirming the presence of stroke symptoms (neurologic deficits)
-
Distinguishing stroke from stroke mimics
-
Establishing a neurologic baseline, should the patient's condition improve or deteriorate
-
Establishing stroke severity, using a structured neurologic exam and score (National Institutes of Health Stroke Scale [NIHSS]) to assist in prognosis and therapeutic selection
Essential components of the neurologic examination include the following evaluations:
-
Cranial nerves
-
Motor function
-
Sensory function
-
Cerebellar function
-
Gait
-
Deep tendon reflexes
-
Language (expressive and receptive capabilities)
-
Mental status and level of consciousness
The skull and spine also should be examined, and signs of meningismus should be sought.
See Clinical Presentation for more detail.
Diagnosis
Emergent brain imaging is essential for evaluation of acute ischemic stroke. Noncontrast computed tomography (CT) scanning is the most commonly used form of neuroimaging in the acute evaluation of patients with apparent acute stroke. The following neuroimaging techniques may also be used emergently:
-
CT angiography and CT perfusion scanning
-
Magnetic resonance imaging (MRI)
-
Carotid duplex scanning
-
Digital subtraction angiography
Lumbar puncture
A lumbar puncture is required to rule out meningitis or subarachnoid hemorrhage when the CT scan is negative but the clinical suspicion remains high
Laboratory studies
Laboratory tests performed in the diagnosis and evaluation of ischemic stroke include the following:
-
Complete blood count (CBC): A baseline study that may reveal a cause for the stroke (eg, polycythemia, thrombocytosis, leukemia), provide evidence of concurrent illness, and ensure absence of thrombocytopenia when considering fibrinolytic therapy
-
Basic chemistry panel: A baseline study that may reveal a stroke mimic (eg, hypoglycemia, hyponatremia) or provide evidence of concurrent illness (eg, diabetes, renal insufficiency)
-
Coagulation studies: May reveal a coagulopathy and are useful when fibrinolytics or anticoagulants are to be used
-
Cardiac biomarkers: Important because of the association of cerebral vascular disease and coronary artery disease
-
Toxicology screening: May assist in identifying intoxicated patients with symptoms/behavior mimicking stroke syndromes or the use of sympathomimetics, which can cause hemorrhagic and ischemic strokes
-
Pregnancy testing: A urine pregnancy test should be obtained for all women of childbearing age with stroke symptoms; recombinant tissue-type plasminogen activator (rt-PA) is a pregnancy class C agent
See Workup for more detail.
Management
The goal for the emergent management of stroke is to complete the following within 60 minutes or less of patient arrival: [2]
-
Assess airway, breathing, and circulation (ABCs) and stabilize the patient as necessary
-
Complete the initial evaluation and assessment, including imaging and laboratory studies
-
Initiate reperfusion therapy, if appropriate
Critical treatment decisions focus on the following:
-
The need for airway management
-
Optimal blood pressure control
-
Identifying potential reperfusion therapies (eg, intravenous fibrinolysis with rt-PA (alteplase) or intra-arterial approaches)
Involvement of a physician with a special interest and training in stroke is ideal. Stroke care units with specially trained nursing and allied healthcare personnel have clearly been shown to improve outcomes.
Ischemic stroke therapies include the following:
-
Fibrinolytic therapy
-
Mechanical thrombectomy
Treatment of comorbid conditions may include the following:
-
Reduce fever
-
Correct hypotension/significant hypertension
-
Correct hypoxia
-
Correct hypoglycemia
-
Manage cardiac arrhythmias
-
Manage myocardial ischemia
Stroke prevention
Primary stroke prevention refers to the treatment of individuals with no previous history of stroke. Measures may include use of the following:
-
Platelet antiaggregants
-
Statins
-
Exercise
-
Lifestyle interventions (eg, smoking cessation, alcohol moderation)
Secondary prevention refers to the treatment of individuals who have already had a stroke. Measures may include use of the following:
-
Platelet antiaggregants
-
Antihypertensives
-
Statins
-
Lifestyle interventions
See Treatment and Medication for more detail.
Background
Acute ischemic stroke (AIS) is characterized by the sudden loss of blood circulation to an area of the brain, typically in a vascular territory, resulting in a corresponding loss of neurologic function. Also previously called cerebrovascular accident (CVA) or stroke syndrome, stroke is a nonspecific state of brain injury with neuronal dysfunction that has several pathophysiologic causes. Strokes can be divided into 2 types: hemorrhagic or ischemic. Acute ischemic stroke is caused by thrombotic or embolic occlusion of a cerebral artery. (See the image below.)
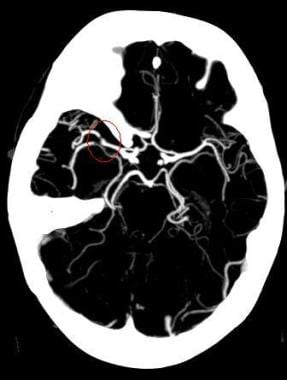
Nearly 800,000 people suffer strokes each year in the United States; 82–92% of these strokes are ischemic. Stroke is the fifth leading cause of adult death and disability, resulting in over $72 billion in annual cost. [5] Between 2012 and 2030, total direct medical stroke-related costs are projected to triple, to $184.1 billion, with the majority of the projected increase in costs arising from those 65 to 79 years of age. [6]
Ischemic and hemorrhagic stroke cannot be reliably differentiated on the basis of clinical examination findings alone. Further evaluation, especially with brain imaging tests (ie, computed tomography [CT] scanning or magnetic resonance imaging [MRI]), is required. (See Workup.)
Stroke categories
The system of categorizing stroke developed in the multicenter Trial of ORG 10172 in Acute Stroke Treatment (TOAST) divides ischemic strokes into the following 3 major subtypes: [3]
-
Large-artery
-
Small-vessel, or lacunar
-
Cardioembolic infarction
Large-artery infarctions often involve thrombotic in situ occlusions on atherosclerotic lesions in the carotid, vertebrobasilar, and cerebral arteries, typically proximal to major branches; however, large-artery infarctions may also be cardioembolic.
Cardiogenic emboli are a common source of recurrent stroke. They may account for up to 20% of acute strokes and have been reported to have the highest 1-month mortality. [7] (See Pathophysiology.)
Small vessel or lacunar strokes are associated with small focal areas of ischemia due to obstruction of single small vessels, typically in deep penetrating arteries, that generate a specific vascular pathology.
In many patients the exact etiology of their stroke is not identified and these are classified as cryptogenic strokes.
Treatment
Recanalization strategies, including intravenous recombinant tissue-type plasminogen activator (alteplase or rt-PA) and intra-arterial approaches, attempt to establish revascularization so that cells within the ischemic penumbra (a metabolically active region, peripheral to the ischemic area, where blood flow is reduced and the cells are potentially viable) can be rescued before irreversible injury occurs. Restoring blood flow can mitigate the effects of ischemia only if performed quickly.
The US Food and Drug Administration (FDA) has approved the use of rt-PA in patients who meet criteria set forth by the National Institute of Neurologic Disorders and Stroke (NINDS). In particular, rt-PA must be given within 3 hours of stroke onset and only after CT scanning has ruled out hemorrhagic stroke.
On the basis of recent European data, the American Heart Association and American Stroke Association recommended expanding the window of treatment from 3 hours to 4.5 hours, with more stringent exclusion criteria for the later period (see Treatment). The FDA has not approved rt-PA for this expanded indication, but this has become the community standard in many institutions.
Other aspects of treatment for acute ischemic stroke include the following:
Optimization of physiologic parameters and prevention of neurologic complications
-
Supplemental oxygen as required (> 94% SaO2)
-
Glycemic control
-
Optimal blood pressure control (with consideration for reperfusion therapies)
-
Prevention of hyperthermia
See also Hemorrhagic Stroke.
Anatomy
The brain is the most metabolically active organ in the body. While representing only 2% of the body's mass, it requires 15–20% of the total resting cardiac output to provide the necessary glucose and oxygen for its metabolism.
Knowledge of cerebrovascular arterial anatomy and the territories supplied by the cerebral arteries is useful in determining which vessels are involved in acute stroke. Atypical patterns of brain ischemia that do not conform to specific vascular distributions may indicate a diagnosis other than ischemic stroke, such as venous infarction.
Arterial distributions
In a simplified model, the cerebral hemispheres are supplied by 3 paired major arteries, specifically, the anterior, middle, and posterior cerebral arteries.
The anterior and middle cerebral arteries carry the anterior circulation and arise from the supraclinoid internal carotid arteries. The anterior cerebral artery (ACA) supplies the medial portion of the frontal and parietal lobes and anterior portions of basal ganglia and anterior internal capsule. (See the image below.)
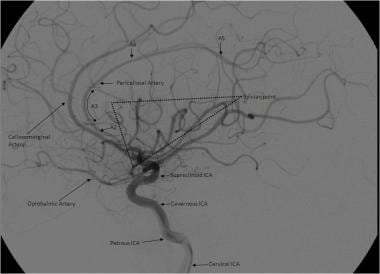
The middle cerebral artery (MCA) supplies the lateral portions of the frontal and parietal lobes, as well as the anterior and lateral portions of the temporal lobes, and gives rise to perforating branches to the globus pallidus, putamen, and internal capsule. The MCA is the dominant source of vascular supply to the hemispheres. (See the images below.)
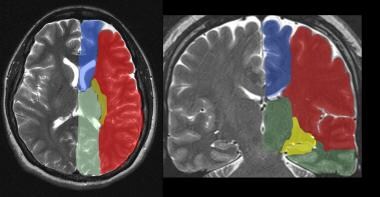
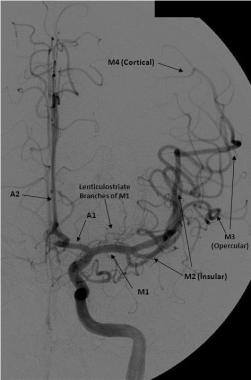
The posterior cerebral arteries arise from the basilar artery and carry the posterior circulation. The posterior cerebral artery (PCA) gives rise to perforating branches that supply the thalami and brainstem and the cortical branches to the posterior and medial temporal lobes and occipital lobes. (See Table 1, below.)
The cerebellar hemispheres are supplied as follows:
-
Inferiorly by the posterior inferior cerebellar artery (PICA), arising from the vertebral artery (see the image below)
Frontal projection from a right vertebral artery angiogram illustrates the posterior circulation. The vertebral arteries join to form the basilar artery. The posterior inferior cerebellar arteries (PICAs) arise from the distal vertebral arteries. The anterior inferior cerebellar arteries (AICAs) arise from the proximal basilar artery. The superior cerebellar arteries (SCAs) arise distally from the basilar artery prior to its bifurcation into the posterior cerebral arteries (PCAs).
-
Superiorly by the superior cerebellar artery
-
Anterolaterally by the anterior inferior cerebellar artery (AICA), from the basilar artery
Table 1. Vascular Supply to the Brain (Open Table in a new window)
VASCULAR TERRITORY |
Structures Supplied |
Anterior Circulation (Carotid) |
|
Anterior Cerebral Artery |
Cortical branches: medial frontal and parietal lobe Medial lenticulostriate branches: caudate head, globus pallidus, anterior limb of internal capsule |
Middle Cerebral Artery |
Cortical branches: lateral frontal and parietal lobes, lateral and anterior temporal lobe Lateral lenticulostriate branches: globus pallidus and putamen, internal capsule |
Anterior Choroidal Artery |
Optic tracts, medial temporal lobe, ventrolateral thalamus, corona radiata, posterior limb of the internal capsule |
Posterior Circulation (Vertebrobasilar) |
|
Posterior Cerebral Artery |
Cortical branches: occipital lobes, medial and posterior temporal and parietal lobes Perforating branches: brainstem, posterior thalamus and midbrain |
Posterior Inferior Cerebellar Artery |
Inferior vermis; posterior and inferior cerebellar hemispheres |
Anterior Inferior Cerebellar Artery |
Anterolateral cerebellum |
Superior Cerebellar Artery |
Superior vermis; superior cerebellum |
Pathophysiology
Acute ischemic strokes result from vascular occlusion secondary to thromboembolic disease (see Etiology). Ischemia causes cell hypoxia and depletion of cellular adenosine triphosphate (ATP). Without ATP, there is no longer the energy to maintain ionic gradients across the cell membrane and cell depolarization. Influx of sodium and calcium ions and passive inflow of water into the cell lead to cytotoxic edema. [8, 9, 10]
Ischemic core and penumbra
An acute vascular occlusion produces heterogeneous regions of ischemia in the affected vascular territory. Local blood flow is limited to any residual flow in the major arterial source plus the collateral supply, if any.
Affected regions with cerebral blood flow of lower than 10 mL/100 g of tissue/min are referred to collectively as the core. These cells are presumed to die within minutes of stroke onset. [11]
Zones of decreased or marginal perfusion (cerebral blood flow < 25 mL/100g of tissue/min) are collectively called the ischemic penumbra. Tissue in the penumbra can remain viable for several hours because of marginal tissue perfusion. [11]
Ischemic cascade
On the cellular level, the ischemic neuron becomes depolarized as ATP is depleted and membrane ion-transport systems fail. Disruption of cellular metabolism also impairs normal sodium-potassium plasma membrane pumps, producing an intracellular increase in sodium, which in turns increases intracellular water content. This cellular swelling is referred to as cytotoxic edema and occurs very early in cerebral ischemia.
Cerebral ischemia impairs the normal sodium-calcium exchange protein also found on cell plasma membranes. The resulting influx of calcium leads to the release of a number of neurotransmitters, including large quantities of glutamate, which in turn activates N-methyl-D-aspartate (NMDA) and other excitatory receptors on other neurons.
These neurons then become depolarized, causing further calcium influx, further glutamate release, and local amplification of the initial ischemic insult. This massive calcium influx also activates various degradative enzymes, leading to the destruction of the cell membrane and other essential neuronal structures. [12] Free radicals, arachidonic acid, and nitric oxide are generated by this process, which leads to further neuronal damage.
Ischemia also directly results in dysfunction of the cerebral vasculature, with breakdown of the blood-brain barrier occurring within 4-6 hours after infarction. Following the barrier’s breakdown, proteins and water flood into the extracellular space, leading to vasogenic edema. This produces greater levels of brain swelling and mass effect that peak at 3–5 days and resolve over the next several weeks with resorption of water and proteins. [13, 14]
Within hours to days after a stroke, specific genes are activated, leading to the formation of cytokines and other factors that, in turn, cause further inflammation and microcirculatory compromise. [12] Ultimately, the ischemic penumbra is consumed by these progressive insults, coalescing with the infarcted core, often within hours of the onset of the stroke.
Infarction results in the death of astrocytes, as well as the supporting oligodendroglial and microglial cells. The infarcted tissue eventually undergoes liquefaction necrosis and is removed by macrophages, with the development of parenchymal volume loss. A well-circumscribed region of cerebrospinal fluid–like low density, resulting from encephalomalacia and cystic change, is eventually seen. The evolution of these chronic changes may be seen in the weeks to months following the infarction. (See the images below.)
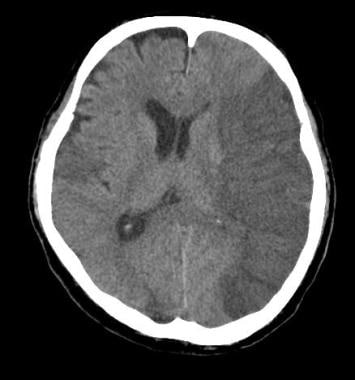
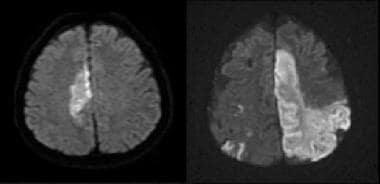
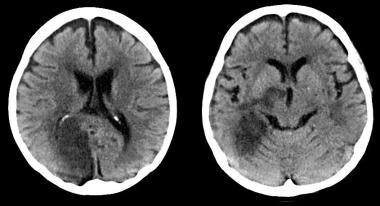
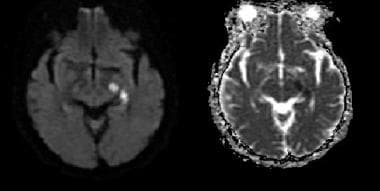
Hemorrhagic transformation of ischemic stroke
Hemorrhagic transformation represents the conversion of an ischemic infarction into an area of hemorrhage. This is estimated to occur in 5% of uncomplicated ischemic strokes, in the absence of fibrinolytic treatment. Hemorrhagic transformation is not always associated with neurologic decline, with the conversion ranging from the development of small petechial hemorrhages to the formation of hematomas that produce neurologic decline and may necessitate surgical evacuation or decompressive hemicraniectomy.
Proposed mechanisms for hemorrhagic transformation include reperfusion of ischemically injured tissue, either from recanalization of an occluded vessel or from collateral blood supply to the ischemic territory or disruption of the blood-brain barrier. With disruption of the blood-brain barrier, red blood cells extravasate from the weakened capillary bed, producing petechial hemorrhage or more frank intraparenchymal hematoma. [8, 15, 16]
Spontaneous hemorrhagic transformation of an ischemic infarct occurs within 2–14 days postictus, usually within the first week. It is more commonly seen following cardioembolic strokes and is more likely to occur with larger infarct volumes. [4, 8, 17] Hemorrhagic transformation is also more likely following administration of rt-PA in patients whose baseline noncontrast CT (NCCT) scans demonstrate areas of hypodensity. [18, 19, 20]
Poststroke cerebral edema and seizures
Although clinically significant cerebral edema can occur after anterior circulation ischemic stroke, it is thought to be somewhat rare (10-20%). [2] Edema and herniation are the most common causes of early death in patients with hemispheric stroke.
Seizures occur in 2-23% of patients within the first days after ischemic stroke. [2] A fraction of patients who have experienced stroke develop chronic seizure disorders.
Etiology
Ischemic strokes result from events that limit or stop blood flow, such as extracranial or intracranial thrombotic embolism, thrombosis in situ, or relative hypoperfusion. As blood flow decreases, neurons cease functioning. Although a range of thresholds has been described, irreversible neuronal ischemia and injury is generally thought to begin at blood flow rates of less than 18 mL/100 g of tissue/min, with cell death occurring rapidly at rates below 10 mL/100 g of tissue/min
Risk factors
Risk factors for ischemic stroke include modifiable and nonmodifiable conditions. Identification of risk factors in each patient can uncover clues to the cause of the stroke and the most appropriate treatment and secondary prevention plan.
Nonmodifiable risk factors include the following (although there are likely many others):
-
Age
-
Race
-
Sex
-
Ethnicity
-
History of migraine headaches [21]
-
Fibromuscular dysplasia
-
Heredity: Family history of stroke or transient ischemic attacks (TIAs)
In a prospective study of 27,860 women aged 45 years or older who were participating in the Women's Health Study, Kurth et al found that migraine with aura was a strong risk factor for any type of stroke. The adjusted incidence of this risk factor per 1000 women per year was similar to those of other known risk factors, including systolic blood pressure 180 mm Hg or higher, body mass index 35 kg/m2 or greater, history of diabetes, family history of myocardial infarction, and smoking. [22]
For migraine with aura, the total incidence of stroke in the study was 4.3 per 1000 women per year, the incidence of ischemic stroke was 3.4 per 1000 per year, and the incidence of hemorrhagic stroke was 0.8 per 1000 per year.
Modifiable risk factors include the following: [23]
-
Hypertension (the most important)
-
Diabetes mellitus
-
Cardiac disease: Atrial fibrillation, valvular disease, heart failure, mitral stenosis, structural anomalies allowing right-to-left shunting (eg, patent foramen ovale), and atrial and ventricular enlargement
-
Hypercholesterolemia
-
Transient ischemic attacks (TIA)
-
Carotid stenosis
-
Hyperhomocystinemia
-
Lifestyle issues: Excessive alcohol intake, tobacco use, illicit drug use, physical inactivity [24]
-
Obesity
-
Oral contraceptive use/postmenopausal hormone use
-
Sickle cell disease
In 2014, the American Heart Association and the American Stroke Association issued guidelines for the reduction of stroke risk specifically in women. These gender-specific recommendations include the following: [25, 26]
-
A stroke risk score should be developed specifically for women
-
Women with a history of high blood pressure before pregnancy should be considered for low-dose aspirin and/or calcium supplement treatment to reduce the risk of preeclampsia
-
Blood pressure medication may be considered for pregnant women with moderately high blood pressure (150-159 mmHg/100-109 mmHg), and pregnant women with severe high blood pressure (160/110 mmHg or above) should be treated
-
Women should be screened for high blood pressure before they start using birth control pills because of an increased risk of stroke
-
Women with migraine headaches with aura should be encouraged to quit smoking to reduce the risk of stroke
-
Women over age 75 should be screened for atrial fibrillation
Genetic and inflammatory mechanisms
Evidence continues to accumulate that inflammation and genetic factors have important roles in the development of atherosclerosis and, specifically, in stroke. According to the current paradigm, atherosclerosis is not a bland cholesterol storage disease, as previously thought, but a dynamic, chronic, inflammatory condition caused by a response to endothelial injury.
Traditional risk factors, such as oxidized low-density lipoprotein (LDL) cholesterol and smoking, contribute to this injury. It has been suggested, however, that infections may also contribute to endothelial injury and atherosclerosis.
Host genetic factors, moreover, may modify the response to these environmental challenges, although inherited risk for stroke is likely multigenic. Even so, specific single-gene disorders with stroke as a component of the phenotype demonstrate the potency of genetics in determining stroke risk.
A number of genes are known to increase susceptibility to ischemic stroke. Mutations to the F2 and F5 genes are relatively common in the general population and increase the risk of thrombosis. Mutations in the following genes also are known to increase the risk of stroke:
-
NOS3: A nitric oxide synthetase gene; involved in vascular relaxation [27]
-
ALOX5AP: Involved in the metabolism of arachidonic acid [28]
-
PRKCH: Involved in major signal transduction systems [29]
Hyperhomocysteinemia and homocystinuria
Hyperhomocysteinemia is implicated in the pathogenesis of ischemic stroke. The most common concern is mutations in the 5,10-methylenetetrahydrofolate reductase (MTHFR) gene. In many populations, the mutant allele frequency reaches polymorphic proportions, and the risk factor for cerebrovascular disease is related to the serum level of homocysteine. Furthermore, in persons who are compound heterozygotes for MTHFR mutation, if elevated homocysteine is found it can be lowered with oral folic acid therapy.
In addition, hyperhomocysteinemia can be seen in cystathione beta synthetase (CBS) deficiency, which is generally referred to as homocystinuria. This disorder is inherited in an autosomal recessive manner. Symptoms usually manifest early in life. Patients have a marfanoid habitus, ectopia lentis, and myopia and generally have intellectual disability. [30]
Thromboembolic events are the most common cause of death for patients with homocystinuria and may be of any type, including myocardial infarction. The risk of having a vascular event in homocystinuria is 50% by age 30. [31] It was previously suggested that persons who are heterozygous for mutations in the CBS gene may have an increased risk of cerebrovascular disease as well, but several more recent studies on this subject failed to replicate this finding.
Amyloid angiopathies
Amyloid angiopathies are also known to increase risk for stroke and dementia. Mutations in the CST3 gene are causative and are inherited in an autosomal dominant manner. Sufferers will have diffuse deposition of amyloid, including in the brain. The onset of symptoms is typically in the third or fourth decade of life, with death occurring before age 60 years. These angiopathies appear to be most common in the Icelandic population. [32]
CADASIL
Cerebral arteriopathy, autosomal dominant, with subcortical infarcts and leukoencephalopathy (CADASIL), is caused by mutations in the NOTCH3 gene. It affects the small arteries of the brain. Strokelike episodes typically occur at a mean age of 46 years, with an age range of 19–67 years. White-matter changes in the brain are typically evident by young adulthood and progress over time. [33]
Migraine headaches occur in 30–40% of people with CADASIL. Approximately 60% of symptomatic individuals have cognitive deficits, which can start as early as age 35 years, and many develop multi-infarct dementia. [34]
Other mutations
Genome-wide association studies have revealed additional loci that are commonly associated with ischemic stroke. Early onset ischemic stroke has been found to be associated with 2 single-nucleotide polymorphisms on 2q23.3. [35]
Large-vessel stroke has been associated with variations in HDAC9, PITX2, and ZFHX3. [36] HDAC9 is located on7p21.1, while PITX2 and ZFHX3 are located on 9p21. It is of note that the 9p21 locus has also been associated with cardiovascular disease.
A polymorphism at 2q36.3 was found in which adenosine substitution conferred a lower risk of ischemic stroke in an additive fashion. [37] An additional study suggested an association between ischemic stroke and a locus on 12p13. [38]
For more information, see Genetic and Inflammatory Mechanisms in Stroke. In addition, complete information on the following metabolic diseases and stroke can be found in the following main articles:
Large-artery occlusion
Large-artery occlusion typically results from embolization of atherosclerotic debris originating from the common or internal carotid arteries or from a cardiac source. A smaller number of large-artery occlusions may arise from plaque ulceration and in situ thrombosis. Large-vessel ischemic strokes more commonly affect the MCA territory, with the ACA territory affected to a lesser degree. (See the images below.)
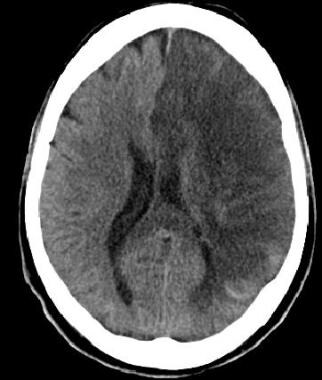
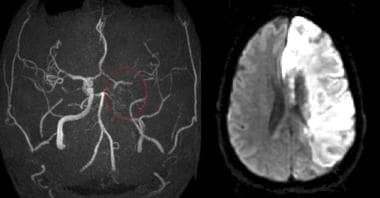
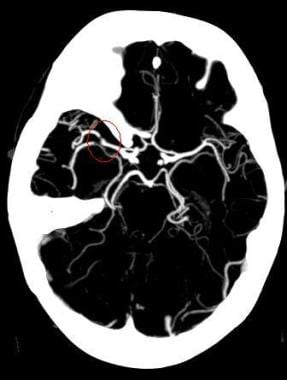
Lacunar strokes
Lacunar strokes represent 13–20% of all ischemic strokes. They result from occlusion of the penetrating branches of the MCA, the lenticulostriate arteries, or the penetrating branches of the circle of Willis, vertebral artery, or basilar artery. The great majority of lacunar strokes are related to hypertension. (See the image below.)
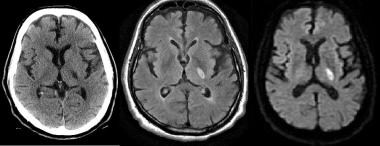
Causes of lacunar infarcts include the following:
-
Microatheroma
-
Lipohyalinosis
-
Fibrinoid necrosis secondary to hypertension or vasculitis
-
Hyaline arteriosclerosis
-
Amyloid angiopathy
-
Microemboli
Embolic strokes
Cardiogenic emboli may account for up to 20% of acute strokes. Emboli may arise from the heart, the extracranial arteries, including the aortic arch or, rarely, the right-sided circulation (paradoxical emboli) with subsequent passage through a patent foramen ovale. [39] Sources of cardiogenic emboli include the following:
-
Valvular thrombi (eg, in mitral stenosis or endocarditis or from use of a prosthetic valve)
-
Mural thrombi (eg, in myocardial infarction, atrial fibrillation, dilated cardiomyopathy, or severe congestive heart failure)
-
Atrial myxoma
Acute myocardial infarction is associated with a 2-3% incidence of embolic strokes, of which 85% occur in the first month after the infarction. [40] Embolic strokes tend to have a sudden onset, and neuroimaging may demonstrate previous infarcts in several vascular territories or may show calcific emboli.
Cardioembolic strokes may be isolated, multiple and in a single hemisphere, or scattered and bilateral; the latter 2 types indicate multiple vascular distributions and are more specific for cardioembolism. Multiple and bilateral infarcts can be the result of embolic showers or recurrent emboli. Other possibilities for single and bilateral hemispheric infarctions include emboli originating from the aortic arch and diffuse thrombotic or inflammatory processes that can lead to multiple small-vessel occlusions. (See the image below.) [41, 42]
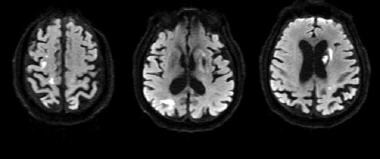
For more information, see Cardioembolic Stroke.
Thrombotic strokes
Thrombogenic factors may include injury to and loss of endothelial cells; this loss exposes the subendothelium and results in platelet activation by the subendothelium, activation of the clotting cascade, inhibition of fibrinolysis, and blood stasis. Thrombotic strokes are generally thought to originate on ruptured atherosclerotic plaques. Arterial stenosis can cause turbulent blood flow, which can promote thrombus formation; atherosclerosis (ie, ulcerated plaques); and platelet adherence. All cause the formation of blood clots that either embolize or occlude the artery.
Intracranial atherosclerosis may be the cause of thrombotic stroke in patients with widespread atherosclerosis. In other patients, especially younger patients, other causes should be considered, including the following: [8, 43]
-
Hypercoagulable states (eg, antiphospholipid antibodies, protein C deficiency, protein S deficiency, pregnancy)
-
Sickle cell disease
-
Fibromuscular dysplasia
-
Arterial dissections
-
Vasoconstriction associated with substance abuse (eg, cocaine, amphetamines)
Watershed infarcts
Vascular watershed, or border-zone, infarctions occur at the most distal areas between arterial territories. They are believed to be secondary to embolic phenomenon or to severe hypoperfusion, as occurs, for example, in carotid occlusion or prolonged hypotension. (See the image below.) [44, 45, 46]
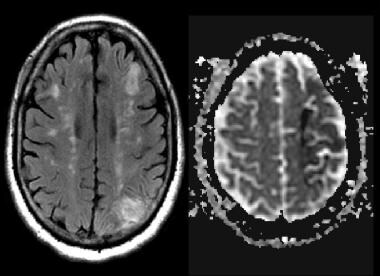
Flow disturbances
Stroke symptoms can result from inadequate cerebral blood flow because of decreased blood pressure (and specifically, decreased cerebral perfusion pressure) or as a result of hematologic hyperviscosity from sickle cell disease or other hematologic illnesses, such as multiple myeloma and polycythemia vera. In these instances, cerebral injury may occur in the presence of damage to other organ systems. For more information, see Blood Dyscrasias and Stroke.
Epidemiology
Stroke is the leading cause of disability and the fifth leading cause of death in the United States. [47, 48] Each year, approximately 795,000 people in the United States experience new (610,000 people) or recurrent (185,000 people) stroke. [5] Epidemiologic studies indicate that 82–92% of strokes in the United States are ischemic.
According to the World Health Organization (WHO), 15 million people suffer stroke worldwide each year. Of these, 5 million die, and another 5 million are left permanently disabled. [49]
Race-, sex-, and age-related demographics
In the United States, Blacks have an age-adjusted risk of death from stroke that is 1.49 times that of Whites. [50] Hispanics have a lower overall incidence of stroke than Whites and Blacks but more frequent lacunar strokes and stroke at an earlier age. Indigenous people in high-income countries have higher incidence of stroke than non-indigenous people. [51]
Men are at higher risk for stroke than women; White men have a stroke incidence of 62.8 per 100,000, with death being the final outcome in 26.3% of cases, while women have a stroke incidence of 59 per 100,000 and a death rate of 39.2%.
Although stroke often is considered a disease of elderly persons, one third of strokes occur in persons younger than 65 years. [48] Risk of stroke increases with age, especially in patients older than 64 years, in whom 75% of all strokes occur.
Prognosis
In the Framingham and Rochester stroke studies, the overall mortality rate at 30 days after stroke was 28%, the mortality rate at 30 days after ischemic stroke was 19%, and the 1-year survival rate for patients with ischemic stroke was 77%. However, the prognosis after acute ischemic stroke varies greatly in individual patients, depending on the stroke severity and on the patient’s premorbid condition, age, and poststroke complications. [3]
A study utilizing the large national Get With The Guidelines - Stroke registry found that the baseline National Institutes of Health Stroke Scale (NIHSS) score was the strongest predictor of early mortality risk, even more so than currently used mortality prediction models incorporating multiple clinical data. [52] Cardiogenic emboli are associated with the highest 1-month mortality in patients with acute stroke.
In late 2018, a new clinical score was developed to identify patients with a high risk of early mortality after an ischemic stroke. Researchers examined data on 77,653 ischemic stroke patients from the Austrian national stroke unit registry, who were treated between 2006 and 2017. They analyzed a comprehensive list of variables on these patients and compared characteristics in patients who died within the first 7 days after the stroke to those who survived. Multivariate analysis was then performed to ascertain which factors were increasingly associated with early stroke death. Key factors included age, stroke severity measured by the National Institutes of Health Stroke Scale (NIHSS), pre-stroke functional disability (modified Rankin Scale > 0), pre-existing heart disease, diabetes mellitus, posterior circulation stroke syndrome, and non-lacunar stroke cause. Results showed that patients with a score ≥ 10 had a 35% risk of dying within the first few days at the stroke unit. [53]
The presence of computed tomography (CT) scan evidence of infarction early in presentation has been associated with poor outcome and with an increased propensity for hemorrhagic transformation after fibrinolytic therapy (see Pathophysiology). [4, 54, 55] Hemorrhagic transformation is estimated to occur in 5% of uncomplicated ischemic strokes in the absence of fibrinolytic therapy, although it is not always associated with neurologic decline. Indeed, hemorrhagic transformation ranges from the development of small petechial hemorrhages to the formation of hematomas requiring evacuation.
Acute ischemic stroke has been associated with acute cardiac dysfunction and arrhythmia, which then correlate with worse functional outcome and morbidity at 3 months. Data suggest that severe hyperglycemia is independently associated with poor outcome and reduced reperfusion in fibrinolysis, as well as extension of the infarcted territory. [56, 57, 58]
In stroke survivors from the Framingham Heart Study, 31% needed help caring for themselves, 20% needed help when walking, and 71% had impaired vocational capacity in long-term follow-up. For more information, see the Medscape Reference article Motor Recovery in Stroke.
Patient Education
Public education must involve all age groups. Incorporating stroke into basic life support (BLS) and cardiopulmonary resuscitation (CPR) curricula is just one way to reach a younger audience. Avenues to reach an audience with a higher stroke risk could include local churches, employers, and senior organizations to promote stroke awareness.
The American Stroke Association (ASA) advises the public to be aware of the symptoms of stroke that are easily recognized, including the sudden onset of any of the following, and to call 911 immediately:
-
Numbness or weakness of face, arm, or leg, especially on 1 side of the body
-
Confusion
-
Difficulty in speaking or understanding
-
Deterioration of vision in 1 or both eyes
-
Difficulty in walking, dizziness, and loss of balance or coordination
-
Severe headache with no known cause
In the spring of 2013, the ASA launched a stroke public education campaign that uses the acronym FAST to teach the warning signs of stroke and the importance of calling 911, as follows:
-
F: Face drooping
-
A: Arm weakness
-
S: Speech difficulty
-
T: Time to call 911
-
Maximum intensity projection (MIP) image from a computed tomography angiogram (CTA) demonstrates a filling defect or high-grade stenosis at the branching point of the right middle cerebral artery (MCA) trunk (red circle), suspicious for thrombus or embolus. CTA is highly accurate in detecting large- vessel stenosis and occlusions, which account for approximately one third of ischemic strokes.
-
Axial noncontrast computed tomography (NCCT) scan demonstrates diffuse hypodensity in the right lentiform nucleus with mass effect upon the frontal horn of the right lateral ventricle in a 70-year-old woman with a history of left-sided weakness for several hours.
-
Magnetic resonance imaging (MRI) scan in a 70-year-old woman with a history of left-sided weakness for several hours. An axial T2 fluid-attenuated inversion recovery (FLAIR) image (left) demonstrates high signal in the lentiform nucleus with mass effect. The axial diffusion-weighted image (middle) demonstrates high signal in the same area, with corresponding low signal on the apparent diffusion coefficient (ADC) maps, consistent with true restricted diffusion and an acute infarction. Maximum intensity projection from a 3-dimensional (3-D) time-of-flight magnetic resonance angiogram (MRA, right) demonstrates occlusion of the distal middle cerebral artery (MCA) trunk (red circle).
-
Cardioembolic stroke: Axial diffusion-weighted images demonstrate scattered foci of high signal in the subcortical and deep white matter bilaterally in a patient with a known cardiac source for embolization. An area of low signal in the left gangliocapsular region may be secondary to prior hemorrhage or subacute to chronic lacunar infarct. Recurrent strokes are most commonly secondary to cardioembolic phenomenon.
-
Axial noncontrast computed tomography (CT) scan demonstrates a focal area of hypodensity in the left posterior limb of the internal capsule in a 60-year-old man with acute onset of right-sided weakness. The lesion demonstrates high signal on the fluid-attenuated inversion recovery (FLAIR) sequence (middle image) and diffusion-weighted magnetic resonance imaging (MRI) scan (right image), with low signal on the apparent diffusion coefficient (ADC) maps indicating an acute lacunar infarction. Lacunar infarcts are typically no more than 1.5 cm in size and can occur in the deep gray matter structures, corona radiata, brainstem, and cerebellum.
-
Magnetic resonance imaging (MRI) scan was obtained in a 62-year-old man with hypertension and diabetes and a history of transient episodes of right-sided weakness and aphasia. The fluid-attenuated inversion recovery (FLAIR) image (left) demonstrates patchy areas of high signal arranged in a linear fashion in the deep white matter, bilaterally. This configuration is typical for deep border-zone, or watershed, infarction, in this case the anterior and posterior middle cerebral artery (MCA) watershed areas. The left-sided infarcts have corresponding low signal on the apparent diffusion coefficient (ADC) map (right), signifying acuity. An old left posterior parietal infarct is noted as well.
-
A 48-year-old man presented with acute left-sided hemiplegia, facial palsy, and right-sided gaze preference. Angiogram with selective injection of the right internal carotid artery demonstrates occlusion of the M1 segment of the right middle cerebral artery (MCA) and A2 segment of the right anterior cerebral artery (ACA; images courtesy of Concentric Medical).
-
Follow-up imaging after mechanical embolectomy in 48-year-old man with acute left-sided hemiplegia, facial palsy, and right-sided gaze preference demonstrates complete recanalization of the right middle cerebral artery (MCA) and partial recanalization of the right A2 segment (images courtesy of Concentric Medical).
-
Cerebral angiogram performed approximately 4.5 hours after symptom onset in a 31-year-old man demonstrates an occlusion of the distal basilar artery (images courtesy of Concentric Medical).
-
Image on the left demonstrates deployment of a clot retrieval device (older generation device) in a 31-year-old man. Followup angiogram after embolectomy demonstrates recanalization of the distal basilar artery with filling of the superior cerebellar arteries and posterior cerebral arteries. The patient had complete resolution of symptoms following embolectomy (images courtesy of Concentric Medical).
-
Noncontrast computed tomography (CT) scan in a 52-year-old man with a history of worsening right-sided weakness and aphasia demonstrates diffuse hypodensity and sulcal effacement with mass effect involving the left anterior and middle cerebral artery territories consistent with acute infarction. There are scattered curvilinear areas of hyperdensity noted suggestive of developing petechial hemorrhage in this large area of infarction.
-
Magnetic resonance angiogram (MRA) in a 52-year-old man demonstrates occlusion of the left precavernous supraclinoid internal carotid artery (ICA, red circle), occlusion or high-grade stenosis of the distal middle cerebral artery (MCA) trunk and attenuation of multiple M2 branches. The diffusion-weighted image (right) demonstrates high signal confirmed to be true restricted diffusion on the apparent diffusion coefficient (ADC) map consistent with acute infarction.
-
Lateral view of a cerebral angiogram illustrates the branches of the anterior cerebral artery (ACA) and Sylvian triangle. The pericallosal artery has been described to arise distal to the anterior communicating artery or distal to the origin of the callosomarginal branch of the ACA. The segmental anatomy of the ACA has been described as follows: the A1 segment extends from the internal carotid artery (ICA) bifurcation to the anterior communicating artery; A2 extends to the junction of the rostrum and genu of the corpus callosum; A3 extends into the bend of the genu of the corpus callosum; A4 and A5 extend posteriorly above the callosal body and superior portion of the splenium. The Sylvian triangle overlies the opercular branches of the middle cerebral artery (MCA), with the apex representing the Sylvian point.
-
Frontal projection from a right vertebral artery angiogram illustrates the posterior circulation. The vertebral arteries join to form the basilar artery. The posterior inferior cerebellar arteries (PICAs) arise from the distal vertebral arteries. The anterior inferior cerebellar arteries (AICAs) arise from the proximal basilar artery. The superior cerebellar arteries (SCAs) arise distally from the basilar artery prior to its bifurcation into the posterior cerebral arteries (PCAs).
-
Frontal view of a cerebral angiogram with selective injection of the left internal carotid artery (ICA) illustrates the anterior circulation. The anterior cerebral artery (ACA) consists of the A1 segment proximal to the anterior communicating artery, with the A2 segment distal to it. The middle cerebral artery (MCA) can be divided into 4 segments: the M1 (horizontal segment) extends to the anterior basal portion of the insular cortex (the limen insulae) and gives off lateral lenticulostriate branches, the M2 (insular segment), M3 (opercular branches), and M4 (distal cortical branches on the lateral hemispheric convexities).
-
Regions of interest are selected for arterial and venous input (image on left) for dynamic susceptibility-weighted perfusion magnetic resonance imaging (MRI). Signal-time curves (image on right) obtained from these regions of interest demonstrate transient signal drop following the administration of intravenous contrast. The information obtained from the dynamic parenchymal signal changes postcontrast is used to generate maps of different perfusion parameters.
-
Vascular distributions: Middle cerebral artery (MCA) infarction. Noncontrast computed tomography (CT) scanning demonstrates a large acute infarction in the MCA territory involving the lateral surfaces of the left frontal, parietal, and temporal lobes, as well as the left insular and subinsular regions, with mass effect and rightward midline shift. There is sparing of the caudate head and at least part of the lentiform nucleus and internal capsule, which receive blood supply from the lateral lenticulostriate branches of the M1 segment of the MCA. Note the lack of involvement of the medial frontal lobe (anterior cerebral artery [ACA] territory), thalami, and paramedian occipital lobe (posterior cerebral artery [PCA] territory).
-
Vascular distributions: Anterior choroidal artery infarction. The diffusion-weighted image (left) demonstrates high signal with associated signal dropout on the apparent diffusion coefficient (ADC) map involving the posterior limb of the internal capsule. This is the typical distribution of the anterior choroidal artery, the last branch of the internal carotid artery (ICA) before bifurcating into the anterior and middle cerebral arteries. The anterior choroidal artery may also arise from the middle cerebral artery (MCA).
-
Vascular distributions: Anterior cerebral artery (ACA) infarction. Diffusion-weighted image on the left demonstrates high signal in the paramedian frontal and high parietal regions. The opposite diffusion-weighted image in a different patient demonstrates restricted diffusion in a larger ACA infarction involving the left paramedian frontal and posterior parietal regions. There is also infarction of the lateral temporoparietal regions bilaterally (both middle cerebral artery [MCA] distributions), greater on the left indicating multivessel involvement and suggesting emboli.
-
Vascular distributions: Posterior cerebral artery (PCA) infarction. The noncontrast computed tomography (CT) images demonstrate PCA distribution infarction involving the right occipital and inferomedial temporal lobes. The image on the right demonstrates additional involvement of the thalamus, also part of the PCA territory.
-
The supratentorial vascular territories of the major cerebral arteries are demonstrated superimposed on axial (left) and coronal (right) T2-weighted images through the level of the basal ganglia and thalami. The middle cerebral artery (MCA; red) supplies the lateral aspects of the hemispheres, including the lateral frontal, parietal, and anterior temporal lobes; insula; and basal ganglia. The anterior cerebral artery (ACA; blue) supplies the medial frontal and parietal lobes. The posterior cerebral artery (PCA; green) supplies the thalami and occipital and inferior temporal lobes. The anterior choroidal artery (yellow) supplies the posterior limb of the internal capsule and part of the hippocampus extending to the anterior and superior surface of the occipital horn of the lateral ventricle.
Tables
What would you like to print?
- Overview
- Presentation
- DDx
- Workup
- Treatment
- Approach Considerations
- Emergency Response and Transport
- Acute Management of Stroke
- Fibrinolytic Therapy
- Intra-arterial Reperfusion
- Antiplatelet Agents
- Blood Pressure Control
- Mechanical Thrombectomy
- Fever Control
- Cerebral Edema Control
- Seizure Control
- Acute Decompensation
- Anticoagulation and Prophylaxis
- Neuroprotective Agents
- Stroke Prevention
- Specialized Stroke Centers
- Consultations
- Diet
- Show All
- Guidelines
- Medication
- Questions & Answers
- Media Gallery
- Tables
- References